Radioprotection of deinococcal exopolysaccharide BRD125 by regenerating hematopoietic stem cells
- PMID: 36226052
- PMCID: PMC9549790
- DOI: 10.3389/fonc.2022.898185
Radioprotection of deinococcal exopolysaccharide BRD125 by regenerating hematopoietic stem cells
Abstract
There is a substantial need for the development of biomaterials for protecting hematopoietic stem cells and enhancing hematopoiesis after radiation damage. Bacterial exopolysaccharide (EPS) has been shown to be very attractive to researchers as a radioprotectant owing to its high antioxidant, anti-cancer, and limited adverse effects. In the present study, we isolated EPS from a novel strain, Deinococcus radiodurans BRD125, which produces EPS in high abundance, and investigated its applicability as a radioprotective biomaterial. We found that EPS isolated from EPS-rich D. radiodurans BRD125 (DeinoPol-BRD125) had an excellent free-radical scavenging effect and reduced irradiation-induced apoptosis. In addition, bone-marrow and spleen-cell apoptosis in irradiated mice were significantly reduced by DeinoPol-BRD125 administration. DeinoPol-BRD125 enhanced the expression of hematopoiesis-related cytokines such as GM-CSF, G-GSF, M-CSF, and SCF, thereby enhancing hematopoietic stem cells protection and regeneration. Taken together, our findings are the first to report the immunological mechanism of a novel radioprotectant, DeinoPol-BRD125, which might constitute an ideal radioprotective and radiation mitigating agent as a supplement drug during radiotherapy.
Keywords: DeinoPol; Deinococcus; exopolysaccharide; hematopoietic stem cell (HSC); mitigator; radiation; radioprotectant; radiotherapy.
Copyright © 2022 Park, Lee, Ji, Lim, Ahn and Seo.
Conflict of interest statement
The authors declare that the research was conducted in the absence of any commercial or financial relationships that could be construed as a potential conflict of interest.
Figures
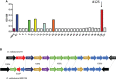
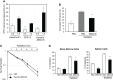
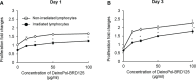
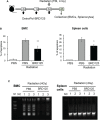
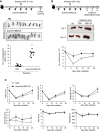
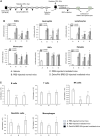
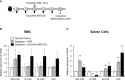
Similar articles
-
Antioxidant Activities of an Exopolysaccharide (DeinoPol) Produced by the Extreme Radiation-Resistant Bacterium Deinococcus radiodurans.Sci Rep. 2020 Jan 9;10(1):55. doi: 10.1038/s41598-019-56141-3. Sci Rep. 2020. PMID: 31919371 Free PMC article.
-
Deinococcus radiodurans Exopolysaccharide Inhibits Staphylococcus aureus Biofilm Formation.Front Microbiol. 2021 Dec 24;12:712086. doi: 10.3389/fmicb.2021.712086. eCollection 2021. Front Microbiol. 2021. PMID: 35002990 Free PMC article.
-
Optimizing and Profiling Prostaglandin E2 as a Medical Countermeasure for the Hematopoietic Acute Radiation Syndrome.Radiat Res. 2021 Feb 1;195(2):115-127. doi: 10.1667/RADE-20-00181.1. Radiat Res. 2021. PMID: 33302300 Free PMC article.
-
Expression of FLT3 receptor and response to FLT3 ligand by leukemic cells.Leukemia. 1996 Apr;10(4):588-99. Leukemia. 1996. PMID: 8618433 Review.
-
MSC-Derived Extracellular Vesicles: New Emergency Treatment to Limit the Development of Radiation-Induced Hematopoietic Syndrome?Health Phys. 2020 Jul;119(1):21-36. doi: 10.1097/HP.0000000000001264. Health Phys. 2020. PMID: 32384375 Review.
Cited by
-
Comparative genomics of Deinococcus radiodurans: unveiling genetic discrepancies between ATCC 13939K and BAA-816 strains.Front Microbiol. 2024 Jun 19;15:1410024. doi: 10.3389/fmicb.2024.1410024. eCollection 2024. Front Microbiol. 2024. PMID: 38962131 Free PMC article.
References
LinkOut - more resources
Full Text Sources
Research Materials