Methylglyoxal and Its Adducts: Induction, Repair, and Association with Disease
- PMID: 36197742
- PMCID: PMC9580021
- DOI: 10.1021/acs.chemrestox.2c00160
Methylglyoxal and Its Adducts: Induction, Repair, and Association with Disease
Abstract
Metabolism is an essential part of life that provides energy for cell growth. During metabolic flux, reactive electrophiles are produced that covalently modify macromolecules, leading to detrimental cellular effects. Methylglyoxal (MG) is an abundant electrophile formed from lipid, protein, and glucose metabolism at intracellular levels of 1-4 μM. MG covalently modifies DNA, RNA, and protein, forming advanced glycation end products (MG-AGEs). MG and MG-AGEs are associated with the onset and progression of many pathologies including diabetes, cancer, and liver and kidney disease. Regulating MG and MG-AGEs is a potential strategy to prevent disease, and they may also have utility as biomarkers to predict disease risk, onset, and progression. Here, we review recent advances and knowledge surrounding MG, including its production and elimination, mechanisms of MG-AGEs formation, the physiological impact of MG and MG-AGEs in disease onset and progression, and the latter in the context of its receptor RAGE. We also discuss methods for measuring MG and MG-AGEs and their clinical application as prognostic biomarkers to allow for early detection and intervention prior to disease onset. Finally, we consider relevant clinical applications and current therapeutic strategies aimed at targeting MG, MG-AGEs, and RAGE to ultimately improve patient outcomes.
Conflict of interest statement
The authors declare no competing financial interest.
Figures
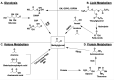
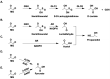
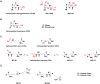
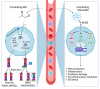
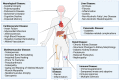
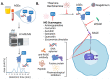
Similar articles
-
Glycative stress from advanced glycation end products (AGEs) and dicarbonyls: An emerging biological factor in cancer onset and progression.Mol Nutr Food Res. 2016 Aug;60(8):1850-64. doi: 10.1002/mnfr.201500759. Epub 2016 Feb 25. Mol Nutr Food Res. 2016. PMID: 26774083 Review.
-
Methylglyoxal - an advanced glycation end products (AGEs) precursor - Inhibits differentiation of human MSC-derived osteoblasts in vitro independently of receptor for AGEs (RAGE).Bone. 2022 Nov;164:116526. doi: 10.1016/j.bone.2022.116526. Epub 2022 Aug 19. Bone. 2022. PMID: 35995334
-
The receptor for advanced glycation end products (RAGE) specifically recognizes methylglyoxal-derived AGEs.Biochemistry. 2014 May 27;53(20):3327-35. doi: 10.1021/bi500046t. Epub 2014 May 13. Biochemistry. 2014. PMID: 24824951 Free PMC article.
-
Advanced Glycation End Products (AGEs), Glutathione and Breast Cancer: Factors, Mechanism and Therapeutic Interventions.Curr Drug Metab. 2019;20(1):65-71. doi: 10.2174/1389200219666180912104342. Curr Drug Metab. 2019. PMID: 30207227
-
Advanced glycation: a novel outlook on atherosclerosis.Curr Pharm Des. 2007;13(36):3681-7. doi: 10.2174/138161207783018608. Curr Pharm Des. 2007. PMID: 18220806 Review.
Cited by
-
Receiver Operator Characteristic (ROC) Analysis of Lipids, Proteins, DNA Oxidative Damage, and Antioxidant Defense in Plasma and Erythrocytes of Young Reproductive-Age Men with Early Stages of Type 1 Diabetes Mellitus (T1DM) Nephropathy in the Irkutsk Region, Russia.Metabolites. 2022 Dec 16;12(12):1282. doi: 10.3390/metabo12121282. Metabolites. 2022. PMID: 36557320 Free PMC article.
-
Pathophysiology of Red Blood Cell Dysfunction in Diabetes and Its Complications.Pathophysiology. 2023 Aug 2;30(3):327-345. doi: 10.3390/pathophysiology30030026. Pathophysiology. 2023. PMID: 37606388 Free PMC article. Review.
-
Identification of Glyoxalase A in Group B Streptococcus and its contribution to methylglyoxal tolerance and virulence.bioRxiv [Preprint]. 2024 Dec 19:2024.07.30.605887. doi: 10.1101/2024.07.30.605887. bioRxiv. 2024. PMID: 39131367 Free PMC article. Preprint.
-
Methylglyoxal-Derived Nucleoside Adducts Drive Vascular Dysfunction in a RAGE-Dependent Manner.Antioxidants (Basel). 2024 Jan 10;13(1):85. doi: 10.3390/antiox13010085. Antioxidants (Basel). 2024. PMID: 38247509 Free PMC article.
-
3D human stem-cell-derived neuronal spheroids for in vitro neurotoxicity testing of methylglyoxal, highly reactive glycolysis byproduct and potent glycating agent.Curr Res Toxicol. 2024 Jun 9;7:100176. doi: 10.1016/j.crtox.2024.100176. eCollection 2024. Curr Res Toxicol. 2024. PMID: 38975063 Free PMC article.
References
-
- Shuck S. C.; Wauchope O. R.; Rose K. L.; Kingsley P. J.; Rouzer C. A.; Shell S. M.; Sugitani N.; Chazin W. J.; Zagol-Ikapitte I.; Boutaud O.; Oates J. A.; Galligan J. J.; Beavers W. N.; Marnett L. J. Protein Modification by Adenine Propenal. Chem. Res. Toxicol. 2014, 27 (10), 1732–1742. 10.1021/tx500218g. - DOI - PMC - PubMed
-
- Beavers W. N.; Rose K. L.; Galligan J. J.; Mitchener M. M.; Rouzer C. A.; Tallman K. A.; Lamberson C. R.; Wang X.; Hill S.; Ivanova P. T.; Brown H. A.; Zhang B.; Porter N. A.; Marnett L. J. Protein Modification by Endogenously Generated Lipid Electrophiles: Mitochondria as the Source and Target. ACS Chem. Biol. 2017, 12 (8), 2062–2069. 10.1021/acschembio.7b00480. - DOI - PMC - PubMed
Publication types
MeSH terms
Substances
LinkOut - more resources
Full Text Sources