Protein conformation and biomolecular condensates
- PMID: 36164646
- PMCID: PMC9508354
- DOI: 10.1016/j.crstbi.2022.09.004
Protein conformation and biomolecular condensates
Abstract
Protein conformation and cell compartmentalization are fundamental concepts and subjects of vast scientific endeavors. In the last two decades, we have witnessed exciting advances that unveiled the conjunction of these concepts. An avalanche of studies highlighted the central role of biomolecular condensates in membraneless subcellular compartmentalization that permits the spatiotemporal organization and regulation of myriads of simultaneous biochemical reactions and macromolecular interactions. These studies have also shown that biomolecular condensation, driven by multivalent intermolecular interactions, is mediated by order-disorder transitions of protein conformation and by protein domain architecture. Conceptually, protein condensation is a distinct level in protein conformational landscape in which collective folding of large collections of molecules takes place. Biomolecular condensates arise by the physical process of phase separation and comprise a variety of bodies ranging from membraneless organelles to liquid condensates to solid-like conglomerates, spanning lengths from mesoscopic clusters (nanometers) to micrometer-sized objects. In this review, we summarize and discuss recent work on the assembly, composition, conformation, material properties, thermodynamics, regulation, and functions of these bodies. We also review the conceptual framework for future studies on the conformational dynamics of condensed proteins in the regulation of cellular processes.
Keywords: Intrinsically disordered proteins; Membraneless organelles; Mesoscopic clusters; Nanocondensates; Phase separation; Protein coacervates; Protein colloids; Protein condensates; Protein conformation; Protein folding.
© 2022 The Authors.
Conflict of interest statement
The authors declare the following financial interests/personal relationships which may be considered as potential competing interests: Mario R. Ermácora reports financial support was provided by 10.13039/501100009559National University of Quilmes. 10.13039/501100002923Conicet, and 10.13039/501100003074Agencia Nacional de Promoción Científica y Tecnológica, Argentina.
Figures
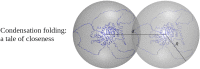
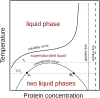
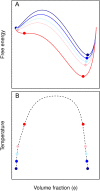
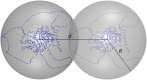
Similar articles
-
Protein nanocondensates: the next frontier.Biophys Rev. 2023 Aug 9;15(4):515-530. doi: 10.1007/s12551-023-01105-1. eCollection 2023 Aug. Biophys Rev. 2023. PMID: 37681092 Free PMC article. Review.
-
Unravelling the microscopic characteristics of intrinsically disordered proteins upon liquid-liquid phase separation.Essays Biochem. 2022 Dec 16;66(7):891-900. doi: 10.1042/EBC20220148. Essays Biochem. 2022. PMID: 36524527 Review.
-
Phase separation in biology and disease-a symposium report.Ann N Y Acad Sci. 2019 Sep;1452(1):3-11. doi: 10.1111/nyas.14126. Epub 2019 Jun 14. Ann N Y Acad Sci. 2019. PMID: 31199001 Free PMC article.
-
Theories for Sequence-Dependent Phase Behaviors of Biomolecular Condensates.Biochemistry. 2018 May 1;57(17):2499-2508. doi: 10.1021/acs.biochem.8b00058. Epub 2018 Mar 13. Biochemistry. 2018. PMID: 29509422 Review.
-
Macromolecular crowding: chemistry and physics meet biology (Ascona, Switzerland, 10-14 June 2012).Phys Biol. 2013 Aug;10(4):040301. doi: 10.1088/1478-3975/10/4/040301. Epub 2013 Aug 2. Phys Biol. 2013. PMID: 23912807
Cited by
-
Protein nanocondensates: the next frontier.Biophys Rev. 2023 Aug 9;15(4):515-530. doi: 10.1007/s12551-023-01105-1. eCollection 2023 Aug. Biophys Rev. 2023. PMID: 37681092 Free PMC article. Review.
-
Condensation of the β-cell secretory granule luminal cargoes pro/insulin and ICA512 RESP18 homology domain.Protein Sci. 2023 Jun;32(6):e4649. doi: 10.1002/pro.4649. Protein Sci. 2023. PMID: 37159024 Free PMC article.
-
Phase separation in cancer at a glance.J Transl Med. 2023 Apr 1;21(1):237. doi: 10.1186/s12967-023-04082-x. J Transl Med. 2023. PMID: 37005672 Free PMC article. Review.
-
The role of biomolecular condensates in protein aggregation.Nat Rev Chem. 2024 Sep;8(9):686-700. doi: 10.1038/s41570-024-00635-w. Epub 2024 Aug 12. Nat Rev Chem. 2024. PMID: 39134696 Review.
-
The pros and cons of ubiquitination on the formation of protein condensates.Acta Biochim Biophys Sin (Shanghai). 2023 Jun 9;55(7):1084-1098. doi: 10.3724/abbs.2023096. Acta Biochim Biophys Sin (Shanghai). 2023. PMID: 37294105 Free PMC article.
References
-
- Adamcik J., Mezzenga R. Amyloid polymorphism in the protein folding and aggregation energy landscape. Angew. Chem. Int. Ed. 2018;57:8370–8382. - PubMed
-
- Alberti S., Hyman A.A. Biomolecular condensates at the nexus of cellular stress, protein aggregation disease and ageing. Nat. Rev. Mol. Cell Biol. 2021;22:196–213. - PubMed
Publication types
LinkOut - more resources
Full Text Sources