Mitochondrial Alterations in Neurons Derived from the Murine AppNL-F Knock-In Model of Alzheimer's Disease
- PMID: 36155507
- PMCID: PMC9697055
- DOI: 10.3233/JAD-220383
Mitochondrial Alterations in Neurons Derived from the Murine AppNL-F Knock-In Model of Alzheimer's Disease
Abstract
Background: Alzheimer's disease (AD) research has relied on mouse models overexpressing human mutant A βPP; however, newer generation knock-in models allow for physiological expression of amyloid-β protein precursor (AβPP) containing familial AD mutations where murine AβPP is edited with a humanized amyloid-β (Aβ) sequence. The AppNL-F mouse model has shown substantial similarities to AD brains developing late onset cognitive impairment.
Objective: In this study, we aimed to characterize mature primary cortical neurons derived from homozygous AppNL-F embryos, especially to identify early mitochondrial alterations in this model.
Methods: Primary cultures of AppNL-F neurons kept in culture for 12-15 days were used to measure Aβ levels, secretase activity, mitochondrial functions, mitochondrial-ER contacts, synaptic function, and cell death.
Results: We detected higher levels of Aβ42 released from AppNL-F neurons as compared to wild-type neurons. AppNL-F neurons, also displayed an increased Aβ42/Aβ40 ratio, similar to adult AppNL-F mouse brain. Interestingly, we found an upregulation in mitochondrial oxygen consumption with concomitant downregulation in glycolytic reserve. Furthermore, AppNL-F neurons were more susceptible to cell death triggered by mitochondrial electron transport chain inhibition. Juxtaposition between ER and mitochondria was found to be substantially upregulated, which may account for upregulated mitochondrial-derived ATP production. However, anterograde mitochondrial movement was severely impaired in this model along with loss in synaptic vesicle protein and impairment in pre- and post-synaptic function.
Conclusion: We show that widespread mitochondrial alterations can be detected in AppNL-F neurons in vitro, where amyloid plaque deposition does not occur, suggesting soluble and oligomeric Aβ-species being responsible for these alterations.
Keywords: Alzheimer’s disease; AppNL-F knock-in mice; mitochondria; mitochondria-ER contact sites; synapses.
Conflict of interest statement
Authors’ disclosures available online (
Figures
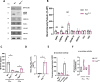
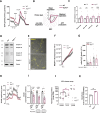
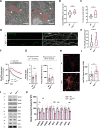
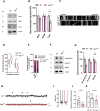
Similar articles
-
Impaired mitochondrial biogenesis, defective axonal transport of mitochondria, abnormal mitochondrial dynamics and synaptic degeneration in a mouse model of Alzheimer's disease.Hum Mol Genet. 2011 Dec 1;20(23):4515-29. doi: 10.1093/hmg/ddr381. Epub 2011 Aug 25. Hum Mol Genet. 2011. PMID: 21873260 Free PMC article.
-
Proteomics Time-Course Study of App Knock-In Mice Reveals Novel Presymptomatic Aβ42-Induced Pathways to Alzheimer's Disease Pathology.J Alzheimers Dis. 2020;75(1):321-335. doi: 10.3233/JAD-200028. J Alzheimers Dis. 2020. PMID: 32280097 Free PMC article.
-
Assessing Sex-Specific Circadian, Metabolic, and Cognitive Phenotypes in the AβPP/PS1 and APPNL-F/NL-F Models of Alzheimer's Disease.J Alzheimers Dis. 2022;85(3):1077-1093. doi: 10.3233/JAD-210629. J Alzheimers Dis. 2022. PMID: 34897085 Free PMC article.
-
Synaptic Mitochondria: An Early Target of Amyloid-β and Tau in Alzheimer's Disease.J Alzheimers Dis. 2021;84(4):1391-1414. doi: 10.3233/JAD-215139. J Alzheimers Dis. 2021. PMID: 34719499 Review.
-
APP transgenic modeling of Alzheimer's disease: mechanisms of neurodegeneration and aberrant neurogenesis.Brain Struct Funct. 2010 Mar;214(2-3):111-26. doi: 10.1007/s00429-009-0232-6. Epub 2009 Nov 29. Brain Struct Funct. 2010. PMID: 20091183 Free PMC article. Review.
Cited by
-
Mitochondrial hypermetabolism precedes impaired autophagy and synaptic disorganization in App knock-in Alzheimer mouse models.Mol Psychiatry. 2023 Sep;28(9):3966-3981. doi: 10.1038/s41380-023-02289-4. Epub 2023 Nov 1. Mol Psychiatry. 2023. PMID: 37907591 Free PMC article.
References
-
- Hardy J, Allsop D (1991) Amyloid deposition as the central event in the aetiology of Alzheimer’s disease. Trends Pharmacol Sci 12, 383–388. - PubMed
Publication types
MeSH terms
Substances
LinkOut - more resources
Full Text Sources
Medical
Research Materials