A feed-forward pathway drives LRRK2 kinase membrane recruitment and activation
- PMID: 36149401
- PMCID: PMC9576273
- DOI: 10.7554/eLife.79771
A feed-forward pathway drives LRRK2 kinase membrane recruitment and activation
Abstract
Activating mutations in the leucine-rich repeat kinase 2 (LRRK2) cause Parkinson's disease, and previously we showed that activated LRRK2 phosphorylates a subset of Rab GTPases (Steger et al., 2017). Moreover, Golgi-associated Rab29 can recruit LRRK2 to the surface of the Golgi and activate it there for both auto- and Rab substrate phosphorylation. Here, we define the precise Rab29 binding region of the LRRK2 Armadillo domain between residues 360-450 and show that this domain, termed 'site #1,' can also bind additional LRRK2 substrates, Rab8A and Rab10. Moreover, we identify a distinct, N-terminal, higher-affinity interaction interface between LRRK2 phosphorylated Rab8 and Rab10 termed 'site #2' that can retain LRRK2 on membranes in cells to catalyze multiple, subsequent phosphorylation events. Kinase inhibitor washout experiments demonstrate that rapid recovery of kinase activity in cells depends on the ability of LRRK2 to associate with phosphorylated Rab proteins, and phosphorylated Rab8A stimulates LRRK2 phosphorylation of Rab10 in vitro. Reconstitution of purified LRRK2 recruitment onto planar lipid bilayers decorated with Rab10 protein demonstrates cooperative association of only active LRRK2 with phospho-Rab10-containing membrane surfaces. These experiments reveal a feed-forward pathway that provides spatial control and membrane activation of LRRK2 kinase activity.
Keywords: LRRK2 kinase; Parkinson's disease; Rab GTPase; biochemistry; cell biology; chemical biology; feed-forward pathway; human; membrane microdomain.
© 2022, Vides et al.
Conflict of interest statement
EV, AA, CC, PL, EP, CL, JS, ES, HD, DA No competing interests declared, SP Senior editor, eLife
Figures
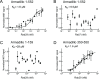
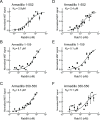
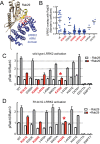
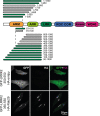
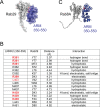
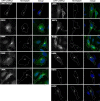
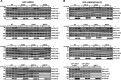
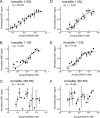
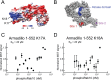
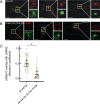
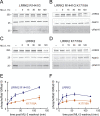
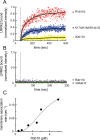
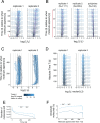
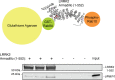
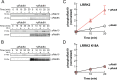
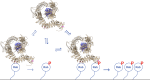
Similar articles
-
Development of phospho-specific Rab protein antibodies to monitor in vivo activity of the LRRK2 Parkinson's disease kinase.Biochem J. 2018 Jan 2;475(1):1-22. doi: 10.1042/BCJ20170802. Biochem J. 2018. PMID: 29127256 Free PMC article.
-
Deciphering the LRRK code: LRRK1 and LRRK2 phosphorylate distinct Rab proteins and are regulated by diverse mechanisms.Biochem J. 2021 Feb 12;478(3):553-578. doi: 10.1042/BCJ20200937. Biochem J. 2021. PMID: 33459343 Free PMC article.
-
Genome-wide screen reveals Rab12 GTPase as a critical activator of Parkinson's disease-linked LRRK2 kinase.Elife. 2023 Oct 24;12:e87098. doi: 10.7554/eLife.87098. Elife. 2023. PMID: 37874635 Free PMC article.
-
LRRK2 and Rab GTPases.Biochem Soc Trans. 2018 Dec 17;46(6):1707-1712. doi: 10.1042/BST20180470. Epub 2018 Nov 22. Biochem Soc Trans. 2018. PMID: 30467121 Review.
-
LRRK2 phosphorylation of Rab GTPases in Parkinson's disease.FEBS Lett. 2023 Mar;597(6):811-818. doi: 10.1002/1873-3468.14492. Epub 2022 Sep 26. FEBS Lett. 2023. PMID: 36114007 Review.
Cited by
-
LRRK2 in Parkinson's disease: upstream regulation and therapeutic targeting.Trends Mol Med. 2024 Oct;30(10):982-996. doi: 10.1016/j.molmed.2024.07.003. Epub 2024 Aug 16. Trends Mol Med. 2024. PMID: 39153957 Review.
-
Inhibition of LRRK2 kinase activity rescues deficits in striatal dopamine physiology in VPS35 p.D620N knock-in mice.NPJ Parkinsons Dis. 2023 Dec 18;9(1):167. doi: 10.1038/s41531-023-00609-7. NPJ Parkinsons Dis. 2023. PMID: 38110354 Free PMC article.
-
Rab29-dependent asymmetrical activation of leucine-rich repeat kinase 2.Science. 2023 Dec 22;382(6677):1404-1411. doi: 10.1126/science.adi9926. Epub 2023 Dec 21. Science. 2023. PMID: 38127736 Free PMC article.
-
Is Glial Dysfunction the Key Pathogenesis of LRRK2-Linked Parkinson's Disease?Biomolecules. 2023 Jan 15;13(1):178. doi: 10.3390/biom13010178. Biomolecules. 2023. PMID: 36671564 Free PMC article. Review.
-
LRRK2 Structure-Based Activation Mechanism and Pathogenesis.Biomolecules. 2023 Mar 28;13(4):612. doi: 10.3390/biom13040612. Biomolecules. 2023. PMID: 37189360 Free PMC article. Review.
References
-
- Adhikari A, Vides E, Pfeffer SR. Reconstitution of LRRK2 membrane recruitment onto planar lipid bilayers V1. Protocols.io. 2022 doi: 10.17504/protocols.io.x54v9y7qzg3e/v1. - DOI
Publication types
MeSH terms
Substances
Associated data
Grants and funding
LinkOut - more resources
Full Text Sources
Research Materials