Intrathecal Injection of the Secretome from ALS Motor Neurons Regulated for miR-124 Expression Prevents Disease Outcomes in SOD1-G93A Mice
- PMID: 36140218
- PMCID: PMC9496075
- DOI: 10.3390/biomedicines10092120
Intrathecal Injection of the Secretome from ALS Motor Neurons Regulated for miR-124 Expression Prevents Disease Outcomes in SOD1-G93A Mice
Abstract
Amyotrophic lateral sclerosis (ALS) is a neurodegenerative disease with short life expectancy and no effective therapy. We previously identified upregulated miR-124 in NSC-34-motor neurons (MNs) expressing human SOD1-G93A (mSOD1) and established its implication in mSOD1 MN degeneration and glial cell activation. When anti-miR-124-treated mSOD1 MN (preconditioned) secretome was incubated in spinal cord organotypic cultures from symptomatic mSOD1 mice, the dysregulated homeostatic balance was circumvented. To decipher the therapeutic potential of such preconditioned secretome, we intrathecally injected it in mSOD1 mice at the early stage of the disease (12-week-old). Preconditioned secretome prevented motor impairment and was effective in counteracting muscle atrophy, glial reactivity/dysfunction, and the neurodegeneration of the symptomatic mSOD1 mice. Deficits in corticospinal function and gait abnormalities were precluded, and the loss of gastrocnemius muscle fiber area was avoided. At the molecular level, the preconditioned secretome enhanced NeuN mRNA/protein expression levels and the PSD-95/TREM2/IL-10/arginase 1/MBP/PLP genes, thus avoiding the neuronal/glial cell dysregulation that characterizes ALS mice. It also prevented upregulated GFAP/Cx43/S100B/vimentin and inflammatory-associated miRNAs, specifically miR-146a/miR-155/miR-21, which are displayed by symptomatic animals. Collectively, our study highlights the intrathecal administration of the secretome from anti-miR-124-treated mSOD1 MNs as a therapeutic strategy for halting/delaying disease progression in an ALS mouse model.
Keywords: ALS mouse model; SOD1-G93A mutation; anti-microRNA-124; intraspinal delivery route; neuroprotection; preservation of motor performance; prevention of glial dysfunction; secretome-based therapy.
Conflict of interest statement
The authors declare no conflict of interest. The funders had no role in the design of the study; in the collection, analyses, or interpretation of data; in the writing of the manuscript; or in the decision to publish the results.
Figures
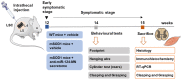

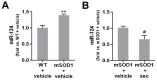
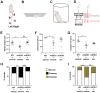
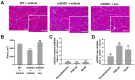
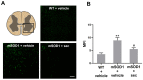
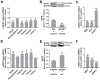
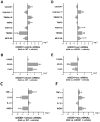
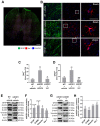
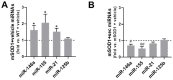
Similar articles
-
Recovery of Depleted miR-146a in ALS Cortical Astrocytes Reverts Cell Aberrancies and Prevents Paracrine Pathogenicity on Microglia and Motor Neurons.Front Cell Dev Biol. 2021 Apr 23;9:634355. doi: 10.3389/fcell.2021.634355. eCollection 2021. Front Cell Dev Biol. 2021. PMID: 33968923 Free PMC article.
-
Exosomes from NSC-34 Cells Transfected with hSOD1-G93A Are Enriched in miR-124 and Drive Alterations in Microglia Phenotype.Front Neurosci. 2017 May 17;11:273. doi: 10.3389/fnins.2017.00273. eCollection 2017. Front Neurosci. 2017. PMID: 28567000 Free PMC article.
-
Overexpression of miR-124 in Motor Neurons Plays a Key Role in ALS Pathological Processes.Int J Mol Sci. 2021 Jun 7;22(11):6128. doi: 10.3390/ijms22116128. Int J Mol Sci. 2021. PMID: 34200161 Free PMC article.
-
Transgenic mice with human mutant genes causing Parkinson's disease and amyotrophic lateral sclerosis provide common insight into mechanisms of motor neuron selective vulnerability to degeneration.Rev Neurosci. 2007;18(2):115-36. doi: 10.1515/revneuro.2007.18.2.115. Rev Neurosci. 2007. PMID: 17593875 Review.
-
CD4 + T Cells and Neuroprotection: Relevance to Motoneuron Injury and Disease.J Neuroimmune Pharmacol. 2015 Dec;10(4):587-94. doi: 10.1007/s11481-015-9625-x. Epub 2015 Jul 7. J Neuroimmune Pharmacol. 2015. PMID: 26148561 Free PMC article. Review.
Cited by
-
SIK2 Controls the Homeostatic Character of the POMC Secretome Acutely in Response to Pharmacological ER Stress Induction.Cells. 2024 Sep 17;13(18):1565. doi: 10.3390/cells13181565. Cells. 2024. PMID: 39329749 Free PMC article.
-
Emerging Role of miR-21-5p in Neuron-Glia Dysregulation and Exosome Transfer Using Multiple Models of Alzheimer's Disease.Cells. 2022 Oct 26;11(21):3377. doi: 10.3390/cells11213377. Cells. 2022. PMID: 36359774 Free PMC article.
-
Exploratory Analysis of MicroRNA Alterations in a Neurodevelopmental Mouse Model for Autism Spectrum Disorder and Schizophrenia.Int J Mol Sci. 2024 Feb 28;25(5):2786. doi: 10.3390/ijms25052786. Int J Mol Sci. 2024. PMID: 38474035 Free PMC article.
-
Spatiotemporal Dysregulation of Neuron-Glia Related Genes and Pro-/Anti-Inflammatory miRNAs in the 5xFAD Mouse Model of Alzheimer's Disease.Int J Mol Sci. 2024 Aug 31;25(17):9475. doi: 10.3390/ijms25179475. Int J Mol Sci. 2024. PMID: 39273422 Free PMC article.
-
Respiratory pathology in the TDP-43 transgenic mouse model of amyotrophic lateral sclerosis.Front Physiol. 2024 Aug 27;15:1430875. doi: 10.3389/fphys.2024.1430875. eCollection 2024. Front Physiol. 2024. PMID: 39403566 Free PMC article.
References
Grants and funding
- ELA-2015-002/Santa Casa da Misericórdia de Lisboa
- PTDC/MED-NEU/31395/2017, UIDB/UIDP/04138/2020 and UID/DTP/04138/2019-2020/Fundação para a Ciência e Tecnologia
- 01-0145-FEDER-031395/Programa Operacional Regional de Lisboa and the Programa Operacional Competitividade e Internacionalização LISBOA
- HR21-00931/La Caixa Foundation and Francisco Luzón Foundation
- PPBI-POCI-01-0145-FEDER-022122/ICVS Scientific Microscopy Platform, member of the national infrastructure PPBI - Portuguese Platform of Bioimaging
LinkOut - more resources
Full Text Sources
Molecular Biology Databases
Research Materials
Miscellaneous