Primary and metastatic tumors exhibit systems-level differences in dependence on mitochondrial respiratory function
- PMID: 36137002
- PMCID: PMC9498964
- DOI: 10.1371/journal.pbio.3001753
Primary and metastatic tumors exhibit systems-level differences in dependence on mitochondrial respiratory function
Abstract
The Warburg effect, aerobic glycolysis, is a hallmark feature of cancer cells grown in culture. However, the relative roles of glycolysis and respiratory metabolism in supporting in vivo tumor growth and processes such as tumor dissemination and metastatic growth remain poorly understood, particularly on a systems level. Using a CRISPRi mini-library enriched for mitochondrial ribosomal protein and respiratory chain genes in multiple human lung cancer cell lines, we analyzed in vivo metabolic requirements in xenograft tumors grown in distinct anatomic contexts. While knockdown of mitochondrial ribosomal protein and respiratory chain genes (mito-respiratory genes) has little impact on growth in vitro, tumor cells depend heavily on these genes when grown in vivo as either flank or primary orthotopic lung tumor xenografts. In contrast, respiratory function is comparatively dispensable for metastatic tumor growth. RNA-Seq and metabolomics analysis of tumor cells expressing individual sgRNAs against mito-respiratory genes indicate overexpression of glycolytic genes and increased sensitivity of glycolytic inhibition compared to control when grown in vitro, but when grown in vivo as primary tumors these cells down-regulate glycolytic mechanisms. These studies demonstrate that discrete perturbations of mitochondrial respiratory chain function impact in vivo tumor growth in a context-specific manner with differential impacts on primary and metastatic tumors.
Conflict of interest statement
The authors have declared that no competing interests exist.
Figures
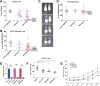
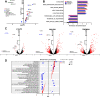
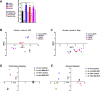
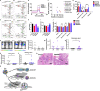
Comment in
-
The requirement for mitochondrial respiration in cancer varies with disease stage.PLoS Biol. 2022 Sep 23;20(9):e3001800. doi: 10.1371/journal.pbio.3001800. eCollection 2022 Sep. PLoS Biol. 2022. PMID: 36149877 Free PMC article.
Similar articles
-
Oxygen consumption can regulate the growth of tumors, a new perspective on the Warburg effect.PLoS One. 2009 Sep 15;4(9):e7033. doi: 10.1371/journal.pone.0007033. PLoS One. 2009. PMID: 19753307 Free PMC article.
-
Mitochondrial metabolism in cancer metastasis: visualizing tumor cell mitochondria and the "reverse Warburg effect" in positive lymph node tissue.Cell Cycle. 2012 Apr 1;11(7):1445-54. doi: 10.4161/cc.19841. Epub 2012 Apr 1. Cell Cycle. 2012. PMID: 22395432 Free PMC article.
-
Mitochondrial oxidative stress in cancer-associated fibroblasts drives lactate production, promoting breast cancer tumor growth: understanding the aging and cancer connection.Cell Cycle. 2011 Dec 1;10(23):4065-73. doi: 10.4161/cc.10.23.18254. Epub 2011 Dec 1. Cell Cycle. 2011. PMID: 22129993 Free PMC article.
-
Decoding Warburg's hypothesis: tumor-related mutations in the mitochondrial respiratory chain.Oncotarget. 2015 Dec 8;6(39):41582-99. doi: 10.18632/oncotarget.6057. Oncotarget. 2015. PMID: 26462158 Free PMC article. Review.
-
The Warburg effect in tumor progression: mitochondrial oxidative metabolism as an anti-metastasis mechanism.Cancer Lett. 2015 Jan 28;356(2 Pt A):156-64. doi: 10.1016/j.canlet.2014.04.001. Epub 2014 Apr 13. Cancer Lett. 2015. PMID: 24732809 Free PMC article. Review.
Cited by
-
The requirement for mitochondrial respiration in cancer varies with disease stage.PLoS Biol. 2022 Sep 23;20(9):e3001800. doi: 10.1371/journal.pbio.3001800. eCollection 2022 Sep. PLoS Biol. 2022. PMID: 36149877 Free PMC article.
-
The oncogenic axis YAP/MYC/EZH2 impairs PTEN tumor suppression activity enhancing lung tumorigenicity.Cell Death Discov. 2024 Oct 25;10(1):452. doi: 10.1038/s41420-024-02216-8. Cell Death Discov. 2024. PMID: 39455556 Free PMC article.
-
Energy metabolism as the hub of advanced non-small cell lung cancer management: a comprehensive view in the framework of predictive, preventive, and personalized medicine.EPMA J. 2024 Apr 8;15(2):289-319. doi: 10.1007/s13167-024-00357-5. eCollection 2024 Jun. EPMA J. 2024. PMID: 38841622 Free PMC article. Review.
References
Publication types
MeSH terms
Substances
Grants and funding
LinkOut - more resources
Full Text Sources
Medical