An Unexpected Encounter: Respiratory Syncytial Virus Nonstructural Protein 1 Interacts with Mediator Subunit MED25
- PMID: 36102648
- PMCID: PMC9555202
- DOI: 10.1128/jvi.01297-22
An Unexpected Encounter: Respiratory Syncytial Virus Nonstructural Protein 1 Interacts with Mediator Subunit MED25
Abstract
Human respiratory syncytial virus (RSV) is the leading cause of severe acute lower respiratory tract infections in infants worldwide. Nonstructural protein NS1 of RSV modulates the host innate immune response by acting as an antagonist of type I and type III interferon (IFN) production and signaling in multiple ways. Likely, NS1 performs this function by interacting with different host proteins. In order to obtain a comprehensive overview of the NS1 interaction partners, we performed three complementary protein-protein interaction screens, i.e., BioID, MAPPIT, and KISS. To closely mimic a natural infection, the BioID proximity screen was performed using a recombinant RSV in which the NS1 protein is fused to a biotin ligase. Remarkably, MED25, a subunit of the Mediator complex, was identified in all three performed screening methods as a potential NS1-interacting protein. We confirmed the interaction between MED25 and RSV NS1 by coimmunoprecipitation, not only upon overexpression of NS1 but also with endogenous NS1 during RSV infection. We also demonstrate that the replication of RSV can be enhanced in MED25 knockout A549 cells, suggesting a potential antiviral role of MED25 during RSV infection. Mediator subunits function as transcriptional coactivators and are involved in transcriptional regulation of their target genes. Therefore, the interaction between RSV NS1 and cellular MED25 might be beneficial for RSV during infection by affecting host transcription and the host immune response to infection. IMPORTANCE Innate immune responses, including the production of type I and III interferons, play a crucial role in the first line of defense against RSV infection. However, only a poor induction of type I IFNs is observed during RSV infection, suggesting that RSV has evolved mechanisms to prevent type I IFN expression by the infected host cell. A unique RSV protein, NS1, is largely responsible for this effect, probably through interaction with multiple host proteins. A better understanding of the interactions that occur between RSV NS1 and host proteins may help to identify targets for an effective antiviral therapy. We addressed this question by performing three complementary protein-protein interaction screens and identified MED25 as an RSV NS1-interacting protein. We propose a role in innate anti-RSV defense for this Mediator complex subunit.
Keywords: MED25; interferon; nonstructural protein; protein-protein interactions; respiratory syncytial virus.
Conflict of interest statement
The authors declare no conflict of interest.
Figures
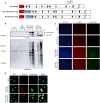
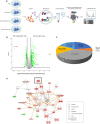
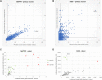
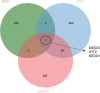
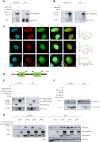
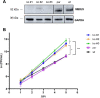
Similar articles
-
Respiratory syncytial virus nonstructural proteins 1 and 2: Exceptional disrupters of innate immune responses.PLoS Pathog. 2019 Oct 17;15(10):e1007984. doi: 10.1371/journal.ppat.1007984. eCollection 2019 Oct. PLoS Pathog. 2019. PMID: 31622448 Free PMC article. Review.
-
Respiratory Syncytial Virus NS1 Protein Targets the Transactivator Binding Domain of MED25.J Mol Biol. 2022 Oct 15;434(19):167763. doi: 10.1016/j.jmb.2022.167763. Epub 2022 Jul 28. J Mol Biol. 2022. PMID: 35907573
-
Nuclear-localized human respiratory syncytial virus NS1 protein modulates host gene transcription.Cell Rep. 2021 Oct 12;37(2):109803. doi: 10.1016/j.celrep.2021.109803. Cell Rep. 2021. PMID: 34644581 Free PMC article.
-
Respiratory syncytial virus NS1 inhibits anti-viral Interferon-α-induced JAK/STAT signaling, by limiting the nuclear translocation of STAT1.Front Immunol. 2024 Jun 13;15:1395809. doi: 10.3389/fimmu.2024.1395809. eCollection 2024. Front Immunol. 2024. PMID: 38938568 Free PMC article.
-
How RSV Proteins Join Forces to Overcome the Host Innate Immune Response.Viruses. 2022 Feb 17;14(2):419. doi: 10.3390/v14020419. Viruses. 2022. PMID: 35216012 Free PMC article. Review.
Cited by
-
Antagonism between viral infection and innate immunity at the single-cell level.PLoS Pathog. 2023 Sep 5;19(9):e1011597. doi: 10.1371/journal.ppat.1011597. eCollection 2023 Sep. PLoS Pathog. 2023. PMID: 37669278 Free PMC article.
-
Virus-Host Protein Interaction Network of the Hepatitis E Virus ORF2-4 by Mammalian Two-Hybrid Assays.Viruses. 2023 Dec 12;15(12):2412. doi: 10.3390/v15122412. Viruses. 2023. PMID: 38140653 Free PMC article.
-
Biochemistry of the Respiratory Syncytial Virus L Protein Embedding RNA Polymerase and Capping Activities.Viruses. 2023 Jan 25;15(2):341. doi: 10.3390/v15020341. Viruses. 2023. PMID: 36851554 Free PMC article. Review.
References
-
- Nair H, Nokes DJ, Gessner BD, Dherani M, Madhi SA, Singleton RJ, O'Brien KL, Roca A, Wright PF, Bruce N, Chandran A, Theodoratou E, Sutanto A, Sedyaningsih ER, Ngama M, Munywoki PK, Kartasasmita C, Simões EAF, Rudan I, Weber MW, Campbell H. 2010. Global burden of acute lower respiratory infections due to respiratory syncytial virus in young children: a systematic review and meta-analysis. Lancet 375:1545–1555. 10.1016/S0140-6736(10)60206-1. - DOI - PMC - PubMed
-
- Shi T, McAllister DA, O'Brien KL, Simoes EAF, Madhi SA, Gessner BD, Polack FP, Balsells E, Acacio S, Aguayo C, Alassani I, Ali A, Antonio M, Awasthi S, Awori JO, Azziz-Baumgartner E, Baggett HC, Baillie VL, Balmaseda A, Barahona A, Basnet S, Bassat Q, Basualdo W, Bigogo G, Bont L, Breiman RF, Brooks WA, Broor S, Bruce N, Bruden D, Buchy P, Campbell S, Carosone-Link P, Chadha M, Chipeta J, Chou M, Clara W, Cohen C, de Cuellar E, Dang D-A, Dash-Yandag B, Deloria-Knoll M, Dherani M, Eap T, Ebruke BE, Echavarria M, de Freitas Lázaro Emediato CC, Fasce RA, Feikin DR, Feng L, RSV Global Epidemiology Network , et al.. 2017. Global, regional, and national disease burden estimates of acute lower respiratory infections due to respiratory syncytial virus in young children in 2015: a systematic review and modelling study. Lancet 390:946–958. 10.1016/S0140-6736(17)30938-8. - DOI - PMC - PubMed
-
- Li Y, Wang X, Blau DM, Caballero MT, Feikin DR, Gill CJ, Madhi SA, Omer SB, Simões EAF, Campbell H, Pariente AB, Bardach D, Bassat Q, Casalegno J-S, Chakhunashvili G, Crawford N, Danilenko D, Do LAH, Echavarria M, Gentile A, Gordon A, Heikkinen T, Huang QS, Jullien S, Krishnan A, Lopez EL, Markić J, Mira-Iglesias A, Moore HC, Moyes J, Mwananyanda L, Nokes DJ, Noordeen F, Obodai E, Palani N, Romero C, Salimi V, Satav A, Seo E, Shchomak Z, Singleton R, Stolyarov K, Stoszek SK, von Gottberg A, Wurzel D, Yoshida L-M, Yung CF, Zar HJ, Nair H, RESCEU Investigators . 2022. Global, regional, and national disease burden estimates of acute lower respiratory infections due to respiratory syncytial virus in children younger than 5 years in 2019: a systematic analysis. Lancet 399:2047–2064. 10.1016/S0140-6736(22)00478-0. - DOI - PMC - PubMed
Publication types
MeSH terms
Substances
LinkOut - more resources
Full Text Sources
Medical
Research Materials