Cryo-EM structure of disease-related prion fibrils provides insights into seeding barriers
- PMID: 36097290
- PMCID: PMC9639217
- DOI: 10.1038/s41594-022-00833-4
Cryo-EM structure of disease-related prion fibrils provides insights into seeding barriers
Abstract
One of the least understood aspects of prion diseases is the structure of infectious prion protein aggregates. Here we report a high-resolution cryo-EM structure of amyloid fibrils formed by human prion protein with the Y145Stop mutation that is associated with a familial prion disease. This structural insight allows us not only to explain previous biochemical findings, but also provides direct support for the conformational adaptability model of prion transmissibility barriers.
© 2022. The Author(s), under exclusive licence to Springer Nature America, Inc.
Conflict of interest statement
Competing Interests
The authors declare no competing interests.
Figures
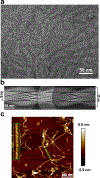
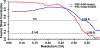
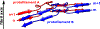
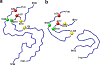
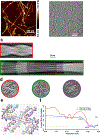
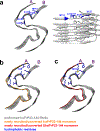
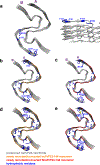
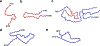
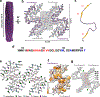
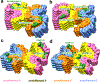
Similar articles
-
PrP P102L and Nearby Lysine Mutations Promote Spontaneous In Vitro Formation of Transmissible Prions.J Virol. 2017 Oct 13;91(21):e01276-17. doi: 10.1128/JVI.01276-17. Print 2017 Nov 1. J Virol. 2017. PMID: 28835493 Free PMC article.
-
The Positively Charged Cluster in the N-terminal Disordered Region may Affect Prion Protein Misfolding: Cryo-EM Structure of Hamster PrP(23-144) Fibrils.J Mol Biol. 2024 Jun 1;436(11):168576. doi: 10.1016/j.jmb.2024.168576. Epub 2024 Apr 18. J Mol Biol. 2024. PMID: 38641239
-
Amyloid fibrils from the N-terminal prion protein fragment are infectious.Proc Natl Acad Sci U S A. 2016 Nov 29;113(48):13851-13856. doi: 10.1073/pnas.1610716113. Epub 2016 Nov 14. Proc Natl Acad Sci U S A. 2016. PMID: 27849581 Free PMC article.
-
The structural line between prion and "prion-like": Insights from prion protein and tau.Curr Opin Neurobiol. 2024 Jun;86:102857. doi: 10.1016/j.conb.2024.102857. Epub 2024 Mar 15. Curr Opin Neurobiol. 2024. PMID: 38489865 Free PMC article. Review.
-
Prion strains viewed through the lens of cryo-EM.Cell Tissue Res. 2023 Apr;392(1):167-178. doi: 10.1007/s00441-022-03676-z. Epub 2022 Aug 27. Cell Tissue Res. 2023. PMID: 36028585 Free PMC article. Review.
Cited by
-
Cryo-EM structures of prion protein filaments from Gerstmann-Sträussler-Scheinker disease.Acta Neuropathol. 2022 Sep;144(3):509-520. doi: 10.1007/s00401-022-02461-0. Epub 2022 Jul 12. Acta Neuropathol. 2022. PMID: 35819518 Free PMC article.
-
Structural biology of ex vivo mammalian prions.J Biol Chem. 2022 Aug;298(8):102181. doi: 10.1016/j.jbc.2022.102181. Epub 2022 Jun 23. J Biol Chem. 2022. PMID: 35752366 Free PMC article. Review.
-
Structural consequences of sequence variation in mammalian prion β2α2 loop segments.Front Neurosci. 2022 Oct 20;16:960322. doi: 10.3389/fnins.2022.960322. eCollection 2022. Front Neurosci. 2022. PMID: 36389229 Free PMC article.
-
Development of a methodology for large-scale production of prions for biological and structural studies.Front Mol Biosci. 2023 Aug 10;10:1184029. doi: 10.3389/fmolb.2023.1184029. eCollection 2023. Front Mol Biosci. 2023. PMID: 37635939 Free PMC article.
-
Pathogenic prion structures at high resolution.PLoS Pathog. 2022 Jun 30;18(6):e1010594. doi: 10.1371/journal.ppat.1010594. eCollection 2022 Jun. PLoS Pathog. 2022. PMID: 35771767 Free PMC article. No abstract available.
References
-
- Kitamoto T, Iizuka R & Tateishi J An amber mutation of prion protein in gerstmann-sträussler syndrome with mutant PrP plaques. Biochemical and Biophysical Research Communications 192, 525–531 (1993). - PubMed
References (to Methods)
-
- Morillas M, Swietnicki W, Gambetti P & Surewicz WK Membrane environment alters the conformational structure of the recombinant human prion protein. Journal of Biological Chemistry 274, 36859–36865 (1999). - PubMed
-
- Mastronarde DN Automated electron microscope tomography using robust prediction of specimen movements. Journal of Structural Biology 152, 36–51 (2005). - PubMed
Publication types
MeSH terms
Substances
Grants and funding
LinkOut - more resources
Full Text Sources