Chronic delta-9-tetrahydrocannabinol (THC) treatment counteracts SIV-induced modulation of proinflammatory microRNA cargo in basal ganglia-derived extracellular vesicles
- PMID: 36096938
- PMCID: PMC9469539
- DOI: 10.1186/s12974-022-02586-9
Chronic delta-9-tetrahydrocannabinol (THC) treatment counteracts SIV-induced modulation of proinflammatory microRNA cargo in basal ganglia-derived extracellular vesicles
Abstract
Background: Early invasion of the central nervous system (CNS) by human immunodeficiency virus (HIV) (Gray et al. in Brain Pathol 6:1-15, 1996; An et al. in Ann Neurol 40:611-6172, 1996), results in neuroinflammation, potentially through extracellular vesicles (EVs) and their micro RNAs (miRNA) cargoes (Sharma et al. in FASEB J 32:5174-5185, 2018; Hu et al. in Cell Death Dis 3:e381, 2012). Although the basal ganglia (BG) is a major target and reservoir of HIV in the CNS (Chaganti et al. in Aids 33:1843-1852, 2019; Mintzopoulos et al. in Magn Reson Med 81:2896-2904, 2019), whether BG produces EVs and the effect of HIV and/or the phytocannabinoid-delta-9-tetrahydrocannabinol (THC) on BG-EVs and HIV neuropathogenesis remain unknown.
Methods: We used the simian immunodeficiency virus (SIV) model of HIV and THC treatment in rhesus macaques (Molina et al. in AIDS Res Hum Retroviruses 27:585-592, 2011) to demonstrate for the first time that BG contains EVs (BG-EVs), and that BG-EVs cargo and function are modulated by SIV and THC. We also used primary astrocytes from the brains of wild type (WT) and CX3CR1+/GFP mice to investigate the significance of BG-EVs in CNS cells.
Results: Significant changes in BG-EV-associated miRNA specific to SIV infection and THC treatment were observed. BG-EVs from SIV-infected rhesus macaques (SIV EVs) contained 11 significantly downregulated miRNAs. Remarkably, intervention with THC led to significant upregulation of 37 miRNAs in BG-EVs (SIV-THC EVs). Most of these miRNAs are predicted to regulate pathways related to inflammation/immune regulation, TLR signaling, Neurotrophin TRK receptor signaling, and cell death/response. BG-EVs activated WT and CX3CR1+/GFP astrocytes and altered the expression of CD40, TNFα, MMP-2, and MMP-2 gene products in primary mouse astrocytes in an EV and CX3CR1 dependent manners.
Conclusions: Our findings reveal a role for BG-EVs as a vehicle with potential to disseminate HIV- and THC-induced changes within the CNS.
Keywords: Astrocytes; Basal ganglia; CX3CR1; Extracellular vesicles; Neuroinflammation; THC.
© 2022. The Author(s).
Conflict of interest statement
The authors report no biomedical financial interests or potential conflicts of interest.
Figures
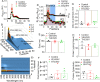
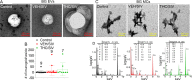
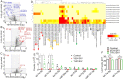
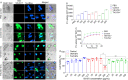
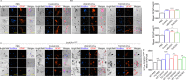
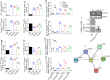
Similar articles
-
Alterations in Abundance and Compartmentalization of miRNAs in Blood Plasma Extracellular Vesicles and Extracellular Condensates during HIV/SIV Infection and Its Modulation by Antiretroviral Therapy (ART) and Delta-9-Tetrahydrocannabinol (Δ9-THC).Viruses. 2023 Feb 24;15(3):623. doi: 10.3390/v15030623. Viruses. 2023. PMID: 36992332 Free PMC article.
-
Long-Term Low-Dose Delta-9-Tetrahydrocannbinol (THC) Administration to Simian Immunodeficiency Virus (SIV) Infected Rhesus Macaques Stimulates the Release of Bioactive Blood Extracellular Vesicles (EVs) that Induce Divergent Structural Adaptations and Signaling Cues.Cells. 2020 Oct 6;9(10):2243. doi: 10.3390/cells9102243. Cells. 2020. PMID: 33036231 Free PMC article.
-
Cannabinoid Attenuation of Intestinal Inflammation in Chronic SIV-Infected Rhesus Macaques Involves T Cell Modulation and Differential Expression of Micro-RNAs and Pro-inflammatory Genes.Front Immunol. 2019 Apr 30;10:914. doi: 10.3389/fimmu.2019.00914. eCollection 2019. Front Immunol. 2019. PMID: 31114576 Free PMC article.
-
Cannabinoid administration attenuates the progression of simian immunodeficiency virus.AIDS Res Hum Retroviruses. 2011 Jun;27(6):585-92. doi: 10.1089/aid.2010.0218. Epub 2010 Nov 23. AIDS Res Hum Retroviruses. 2011. PMID: 20874519 Free PMC article. Review.
-
Plasma neuronal exosomes serve as biomarkers of cognitive impairment in HIV infection and Alzheimer's disease.J Neurovirol. 2019 Oct;25(5):702-709. doi: 10.1007/s13365-018-0695-4. Epub 2019 Jan 4. J Neurovirol. 2019. PMID: 30610738 Free PMC article. Review.
Cited by
-
Antiviral activities of hemp cannabinoids.Clin Sci (Lond). 2023 Apr 26;137(8):633-643. doi: 10.1042/CS20220193. Clin Sci (Lond). 2023. PMID: 37083031 Free PMC article.
-
Effects of Oral Cannabinoids on Systemic Inflammation and Viral Reservoir Markers in People with HIV on Antiretroviral Therapy: Results of the CTN PT028 Pilot Clinical Trial.Cells. 2023 Jul 8;12(14):1811. doi: 10.3390/cells12141811. Cells. 2023. PMID: 37508476 Free PMC article.
-
Comparison of decarboxylation rates of acidic cannabinoids between secretory cavity contents and air-dried inflorescence extracts in Cannabis sativa cv. 'Cherry Wine'.Sci Rep. 2024 Jul 16;14(1):16411. doi: 10.1038/s41598-024-66420-3. Sci Rep. 2024. PMID: 39013926 Free PMC article.
References
-
- Sharma H, Chinnappan M, Agarwal S, Dalvi P, Gunewardena S, O'Brien-Ladner A, Dhillon NK. Macrophage-derived extracellular vesicles mediate smooth muscle hyperplasia: role of altered miRNA cargo in response to HIV infection and substance abuse. FASEB J. 2018;32:5174–5185. doi: 10.1096/fj.201701558R. - DOI - PMC - PubMed
MeSH terms
Substances
Grants and funding
- R33 DA053643/DA/NIDA NIH HHS/United States
- R21 DA053643/DA/NIDA NIH HHS/United States
- P51 OD011133/OD/NIH HHS/United States
- R01 MH123093/MH/NIMH NIH HHS/United States
- R01DA050169/DA/NIDA NIH HHS/United States
- U42 OD010442/OD/NIH HHS/United States
- P51 RR000164/RR/NCRR NIH HHS/United States
- R01MH123093/MH/NIMH NIH HHS/United States
- P30 AI161943/AI/NIAID NIH HHS/United States
- R01MH123093S1/MH/NIMH NIH HHS/United States
- R01 DA042524/DA/NIDA NIH HHS/United States
- R01 DA042348/DA/NIDA NIH HHS/United States
- R01 DA050169/DA/NIDA NIH HHS/United States
- R01DA029718/DA/NIDA NIH HHS/United States
LinkOut - more resources
Full Text Sources
Research Materials
Miscellaneous