Extracellular Vesicle-Mediated miR-150-3p Delivery in Joint Homeostasis: A Potential Treatment for Osteoarthritis?
- PMID: 36078172
- PMCID: PMC9454967
- DOI: 10.3390/cells11172766
Extracellular Vesicle-Mediated miR-150-3p Delivery in Joint Homeostasis: A Potential Treatment for Osteoarthritis?
Abstract
Background: The disruption of joint homeostasis is a critical event during the process of joint injury in osteoarthritis (OA). As regulatory molecules, microRNAs (miRNAs) can be released from secretory cells and delivered to recipient cells through extracellular vesicles (EVs), thereby playing an important role in regulating joint homeostasis. We hypothesized that the fibroblast-like synoviocytes (FLSs) in healthy joints could release EVs enriched in miRNAs that can maintain joint homeostasis by regulating the signal transduction pathways in the joints, whereby the articular cartilage (AC) is protected from degeneration, and OA progression is delayed.
Methods: Via high-throughput sequencing and qPCR, we found that miR-150-3p was enriched in the circulating EVs in healthy rats. Next, we established an in vitro cell model in which chondrocytes were cultured with (i) FLSs transfected with miR-150-3p mimics or (ii) EVs released by FLSs (FLS-EVs) inside the healthy synovial membrane (SM). The transportation mechanism from FLSs to chondrocytes was studied using the EV inhibitor GW4869, and the FLSs were transfected with a miR-150-3p mimic or inhibitor. To assess the therapeutic effect of miR-150-3p-carrying EVs (EVs-150) in vivo, healthy FLS-derived EVs (H-FLS-EVs) were injected into the tail vein of rats with OA at various stages of the pathogenesis and evaluated for the progression of OA.
Results: The chondrocytes could uptake fluorescent-labeled miR-150-3p mimics and FLS-EVs, and GW4869 suppressed this uptake. The overexpression of miR-150-3p could significantly reduce the concentrations of pro-inflammatory cytokines in the cell culture medium and the expression of the miR-150-3p target T cell receptor-interacting molecule 14 (Trim14), as well as the innate immune-related factors, including nuclear factor kappa B (NF-κB) and interferon-β (IFN-β). Similarly to the in vitro findings, the miR-150-3p level in the serum EVs was significantly upregulated among the EV-treated rats. In the AC of the OA rat model injected with H-FLS-EVs, the joint degeneration was suppressed, and Type II collagen (COLII) and aggrecan (ACAN) were significantly upregulated, whereas the innate immune-related factors Trim14, NF-κB, and IFN-β were downregulated compared with the levels in the untreated OA rats. Notably, the suppression of joint degeneration was more significant when H-FLS-EVs were administered at the early stages of OA rather than the late stages.
Conclusion: H-FLS-EVs protect chondrocyte function and maintain joint homeostasis by modulating the innate immune response by suppressing the Trim14/NF-κB/IFNβ axis. These effects are achieved through the EV-mediated transport of miR-150-3p from the FLSs to the chondrocytes. Our findings show that EV-mediated miR-150-3p can be used to suppress OA, thus providing a novel therapeutic strategy. Additionally, the EV-mediated miR-150-3p transport may also serve as a potential biomarker in the diagnosis, treatment, and prognosis of OA.
Keywords: extracellular vesicles; innate immune response; joint homeostasis; miR-150-3p; osteoarthritis.
Conflict of interest statement
The authors have declared that there are no competing interest.
Figures
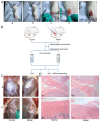
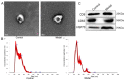
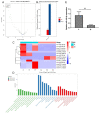
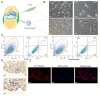
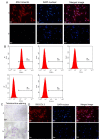
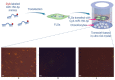
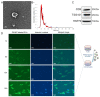
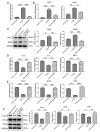
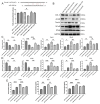
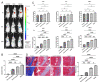
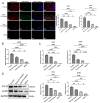
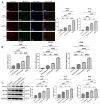
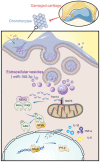
Similar articles
-
Exosomes derived from miR-146a-overexpressing fibroblast-like synoviocytes in cartilage degradation and macrophage M1 polarization: a novel protective agent for osteoarthritis?Front Immunol. 2024 May 23;15:1361606. doi: 10.3389/fimmu.2024.1361606. eCollection 2024. Front Immunol. 2024. PMID: 38846937 Free PMC article.
-
Interaction with hyaluronan matrix and miRNA cargo as contributors for in vitro potential of mesenchymal stem cell-derived extracellular vesicles in a model of human osteoarthritic synoviocytes.Stem Cell Res Ther. 2019 Mar 29;10(1):109. doi: 10.1186/s13287-019-1215-z. Stem Cell Res Ther. 2019. PMID: 30922413 Free PMC article.
-
Synovial mesenchymal stem cell-derived extracellular vesicles alleviate chondrocyte damage during osteoarthritis through microRNA-130b-3p-mediated inhibition of the LRP12/AKT/β-catenin axis.Immunopharmacol Immunotoxicol. 2022 Apr;44(2):247-260. doi: 10.1080/08923973.2022.2038192. Epub 2022 Feb 17. Immunopharmacol Immunotoxicol. 2022. PMID: 35174753
-
Harnessing Tissue-derived Extracellular Vesicles for Osteoarthritis Theranostics.Theranostics. 2022 Jan 1;12(1):207-231. doi: 10.7150/thno.62708. eCollection 2022. Theranostics. 2022. PMID: 34987642 Free PMC article. Review.
-
Extracellular Vesicles and Autophagy in Osteoarthritis.Biomed Res Int. 2016;2016:2428915. doi: 10.1155/2016/2428915. Epub 2016 Dec 18. Biomed Res Int. 2016. PMID: 28078284 Free PMC article. Review.
Cited by
-
Exosomes derived from miR-146a-overexpressing fibroblast-like synoviocytes in cartilage degradation and macrophage M1 polarization: a novel protective agent for osteoarthritis?Front Immunol. 2024 May 23;15:1361606. doi: 10.3389/fimmu.2024.1361606. eCollection 2024. Front Immunol. 2024. PMID: 38846937 Free PMC article.
-
Intraarticular treatment with integrin α10β1-selected mesenchymal stem cells affects microRNA expression in experimental post-traumatic osteoarthritis in horses.Front Vet Sci. 2024 Mar 26;11:1374681. doi: 10.3389/fvets.2024.1374681. eCollection 2024. Front Vet Sci. 2024. PMID: 38596460 Free PMC article.
-
Role of crosstalk between synovial cells and chondrocytes in osteoarthritis (Review).Exp Ther Med. 2024 Mar 15;27(5):201. doi: 10.3892/etm.2024.12490. eCollection 2024 May. Exp Ther Med. 2024. PMID: 38590580 Free PMC article. Review.
-
The Role of Extracellular Vesicles in the Pathogenesis and Treatment of Rheumatoid Arthritis and Osteoarthritis.Cells. 2023 Nov 27;12(23):2716. doi: 10.3390/cells12232716. Cells. 2023. PMID: 38067147 Free PMC article. Review.
-
Recent Advances in Extracellular Vesicles in Amyotrophic Lateral Sclerosis and Emergent Perspectives.Cells. 2023 Jul 1;12(13):1763. doi: 10.3390/cells12131763. Cells. 2023. PMID: 37443797 Free PMC article. Review.
References
-
- Sacitharan P.K. Biochemistry and Cell Biology of Ageing: Part II Clinical Science. Volume 91. Springer; Singapore: 2019. Ageing and Osteoarthritis; pp. 123–159. Subcellular Biochemistry. - PubMed
-
- Hawker G.A. Osteoarthritis is a serious disease. Clin. Exp. Rheumatol. 2019;37((Suppl. 120)):3–6. - PubMed
Publication types
MeSH terms
Substances
Grants and funding
LinkOut - more resources
Full Text Sources
Medical
Research Materials