Utility of NO and H2S donating platforms in managing COVID-19: Rationale and promise
- PMID: 36029975
- PMCID: PMC9398942
- DOI: 10.1016/j.niox.2022.08.003
Utility of NO and H2S donating platforms in managing COVID-19: Rationale and promise
Abstract
Viral infections are a continuing global burden on the human population, underscored by the ramifications of the COVID-19 pandemic. Current treatment options and supportive therapies for many viral infections are relatively limited, indicating a need for alternative therapeutic approaches. Virus-induced damage occurs through direct infection of host cells and inflammation-related changes. Severe cases of certain viral infections, including COVID-19, can lead to a hyperinflammatory response termed cytokine storm, resulting in extensive endothelial damage, thrombosis, respiratory failure, and death. Therapies targeting these complications are crucial in addition to antiviral therapies. Nitric oxide and hydrogen sulfide are two endogenous gasotransmitters that have emerged as key signaling molecules with a broad range of antiviral actions in addition to having anti-inflammatory properties and protective functions in the vasculature and respiratory system. The enhancement of endogenous nitric oxide and hydrogen sulfide levels thus holds promise for managing both early-stage and later-stage viral infections, including SARS-CoV-2. Using SARS-CoV-2 as a model for similar viral infections, here we explore the current evidence regarding nitric oxide and hydrogen sulfide's use to limit viral infection, resolve inflammation, and reduce vascular and pulmonary damage.
Keywords: Antiviral; COVID-19; Cytokine storm; Endothelium; Hydrogen sulfide; Inflammation; Nitric oxide; iNOS.
Copyright © 2022 Elsevier Inc. All rights reserved.
Conflict of interest statement
Declaration of competing interest The authors declare no conflict of interest.
Figures
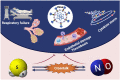
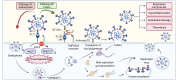
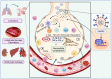
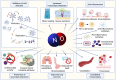
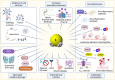
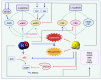
Similar articles
-
Decreased availability of nitric oxide and hydrogen sulfide is a hallmark of COVID-19.Redox Biol. 2021 Jul;43:101982. doi: 10.1016/j.redox.2021.101982. Epub 2021 May 8. Redox Biol. 2021. PMID: 34020311 Free PMC article.
-
Hydrogen Sulfide: Physiological Roles and Therapeutic Implications against COVID-19.Curr Med Chem. 2024;31(21):3132-3148. doi: 10.2174/0929867330666230502111227. Curr Med Chem. 2024. PMID: 37138436 Review.
-
H2S as a potential defense against COVID-19?Am J Physiol Cell Physiol. 2020 Aug 1;319(2):C244-C249. doi: 10.1152/ajpcell.00187.2020. Epub 2020 Jun 9. Am J Physiol Cell Physiol. 2020. PMID: 32515982 Free PMC article. Review.
-
Monoclonal Antibody Therapy For High-Risk Coronavirus (COVID 19) Patients With Mild To Moderate Disease Presentations (Archived).2023 Feb 5. In: StatPearls [Internet]. Treasure Island (FL): StatPearls Publishing; 2024 Jan–. 2023 Feb 5. In: StatPearls [Internet]. Treasure Island (FL): StatPearls Publishing; 2024 Jan–. PMID: 34033365 Free Books & Documents.
-
Hydrogen sulfide: From a toxic gas to a potential therapy for COVID-19 and inflammatory disorders.Nitric Oxide. 2023 Nov 1;140-141:8-15. doi: 10.1016/j.niox.2023.08.002. Epub 2023 Aug 28. Nitric Oxide. 2023. PMID: 37648016 Review.
Cited by
-
Pleiotropic Functions of Nitric Oxide Produced by Ascorbate for the Prevention and Mitigation of COVID-19: A Revaluation of Pauling's Vitamin C Therapy.Microorganisms. 2023 Feb 3;11(2):397. doi: 10.3390/microorganisms11020397. Microorganisms. 2023. PMID: 36838362 Free PMC article. Review.
-
The Renin-Angiotensin-Aldosterone System, Nitric Oxide, and Hydrogen Sulfide at the Crossroads of Hypertension and COVID-19: Racial Disparities and Outcomes.Int J Mol Sci. 2022 Nov 11;23(22):13895. doi: 10.3390/ijms232213895. Int J Mol Sci. 2022. PMID: 36430371 Free PMC article. Review.
-
Hydrogen Sulfide Ameliorates SARS-CoV-2-Associated Lung Endothelial Barrier Disruption.Biomedicines. 2023 Jun 22;11(7):1790. doi: 10.3390/biomedicines11071790. Biomedicines. 2023. PMID: 37509430 Free PMC article.
References
-
- Ryu W.-S. In: Molecular Virology of Human Pathogenic Viruses. Ryu W.-S., editor. Academic Press; Boston: 2017. Chapter 21 - new emerging viruses; pp. 289–302.
-
- B.W.J. Mahy, Emerging and Reemerging Virus Diseases of Vertebrates☆, Reference Module in Biomedical Sciences, Elsevier2014.
-
- Zhu N., Zhang D., Wang W., Li X., Yang B., Song J., Zhao X., Huang B., Shi W., Lu R., Niu P., Zhan F., Ma X., Wang D., Xu W., Wu G., Gao G.F., Tan W., China Novel Coronavirus I., Research T. A novel coronavirus from patients with pneumonia in China, 2019. N. Engl. J. Med. 2020;382(8):727–733. - PMC - PubMed
-
- Santos R., Monteiro S. Epidemiology, control, and prevention of emerging zoonotic viruses. Viruses in Food and Water. 2013:442–457.
Publication types
MeSH terms
Substances
Grants and funding
LinkOut - more resources
Full Text Sources
Medical
Miscellaneous