Conformational Plasticity of Centrin 1 from Toxoplasma gondii in Binding to the Centrosomal Protein SFI1
- PMID: 36009009
- PMCID: PMC9406199
- DOI: 10.3390/biom12081115
Conformational Plasticity of Centrin 1 from Toxoplasma gondii in Binding to the Centrosomal Protein SFI1
Abstract
Centrins are calcium (Ca2+)-binding proteins that are involved in many cellular functions including centrosome regulation. A known cellular target of centrins is SFI1, a large centrosomal protein containing multiple repeats that represent centrin-binding motifs. Recently, a protein homologous to yeast and mammalian SFI1, denominated TgSFI1, which shares SFI1-repeat organization, was shown to colocalize at centrosomes with centrin 1 from Toxoplasma gondii (TgCEN1). However, the molecular details of the interaction between TgCEN1 and TgSFI1 remain largely unknown. Herein, combining different biophysical methods, including isothermal titration calorimetry, nuclear magnetic resonance, circular dichroism, and fluorescence spectroscopy, we determined the binding properties of TgCEN1 and its individual N- and C-terminal domains to synthetic peptides derived from distinct repeats of TgSFI1. Overall, our data indicate that the repeats in TgSFI1 constitute binding sites for TgCEN1, but the binding modes of TgCEN1 to the repeats differ appreciably in terms of binding affinity, Ca2+ sensitivity, and lobe-specific interaction. These results suggest that TgCEN1 displays remarkable conformational plasticity, allowing for the distinct repeats in TgSFI1 to possess precise modes of TgCEN1 binding and regulation during Ca2+ sensing, which appears to be crucial for the dynamic association of TgCEN1 with TgSFI1 in the centrosome architecture.
Keywords: SFI1 protein; Toxoplasma gondii; calcium; centrin; protein-peptide interactions.
Conflict of interest statement
The authors declare no conflict of interest.
Figures
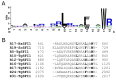
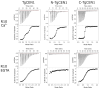
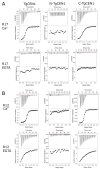
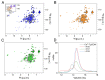
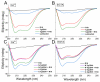
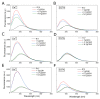
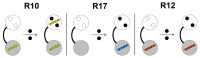
Similar articles
-
The interplay of self-assembly and target binding in centrin 1 from Toxoplasma gondii.Biochem J. 2021 Jul 16;478(13):2571-2587. doi: 10.1042/BCJ20210295. Biochem J. 2021. PMID: 34114596 Free PMC article.
-
Distinct Calcium Binding and Structural Properties of Two Centrin Isoforms from Toxoplasma gondii.Biomolecules. 2020 Aug 4;10(8):1142. doi: 10.3390/biom10081142. Biomolecules. 2020. PMID: 32759683 Free PMC article.
-
Binding of calcium, magnesium, and target peptides to Cdc31, the centrin of yeast Saccharomyces cerevisiae.Biochemistry. 2011 Jul 26;50(29):6409-22. doi: 10.1021/bi200518d. Epub 2011 Jun 29. Biochemistry. 2011. PMID: 21714500
-
Centrosomes: Sfi1p and centrin unravel a structural riddle.Curr Biol. 2004 Jan 6;14(1):R27-9. doi: 10.1016/j.cub.2003.12.019. Curr Biol. 2004. PMID: 14711432 Review.
-
Duplication of the Yeast Spindle Pole Body Once per Cell Cycle.Mol Cell Biol. 2016 Apr 15;36(9):1324-31. doi: 10.1128/MCB.00048-16. Print 2016 May. Mol Cell Biol. 2016. PMID: 26951196 Free PMC article. Review.
Cited by
-
Structural Basis for Cyclosporin Isoform-Specific Inhibition of Cyclophilins from Toxoplasma gondii.ACS Infect Dis. 2023 Feb 10;9(2):365-377. doi: 10.1021/acsinfecdis.2c00566. Epub 2023 Jan 18. ACS Infect Dis. 2023. PMID: 36653744 Free PMC article.
-
An Sfi1-like centrin-interacting centriolar plaque protein affects nuclear microtubule homeostasis.PLoS Pathog. 2023 May 2;19(5):e1011325. doi: 10.1371/journal.ppat.1011325. eCollection 2023 May. PLoS Pathog. 2023. PMID: 37130129 Free PMC article.
-
Evaluating the potential of non-immunosuppressive cyclosporin analogs for targeting Toxoplasma gondii cyclophilin: Insights from structural studies.Protein Sci. 2024 Oct;33(10):e5157. doi: 10.1002/pro.5157. Protein Sci. 2024. PMID: 39312281 Free PMC article.
References
-
- Jani D., Lutz S., Marshall N.J., Fischer T., Kohler A., Ellisdon A.M., Hurt E., Stewart M. Sus1, Cdc31, and the Sac3 CID region form a conserved interaction platform that promotes nuclear pore association and mRNA export. Mol. Cell. 2009;33:727–737. doi: 10.1016/j.molcel.2009.01.033. - DOI - PMC - PubMed
Publication types
MeSH terms
Substances
Grants and funding
LinkOut - more resources
Full Text Sources
Miscellaneous