Organ-On-A-Chip Models of the Blood-Brain Barrier: Recent Advances and Future Prospects
- PMID: 35978444
- PMCID: PMC9529899
- DOI: 10.1002/smll.202201401
Organ-On-A-Chip Models of the Blood-Brain Barrier: Recent Advances and Future Prospects
Abstract
The human brain and central nervous system (CNS) present unique challenges in drug development for neurological diseases. One major obstacle is the blood-brain barrier (BBB), which hampers the effective delivery of therapeutic molecules into the brain while protecting it from blood-born neurotoxic substances and maintaining CNS homeostasis. For BBB research, traditional in vitro models rely upon Petri dishes or Transwell systems. However, these static models lack essential microenvironmental factors such as shear stress and proper cell-cell interactions. To this end, organ-on-a-chip (OoC) technology has emerged as a new in vitro modeling approach to better recapitulate the highly dynamic in vivo human brain microenvironment so-called the neural vascular unit (NVU). Such BBB-on-a-chip models have made substantial progress over the last decade, and concurrently there has been increasing interest in modeling various neurological diseases such as Alzheimer's disease and Parkinson's disease using OoC technology. In addition, with recent advances in other scientific technologies, several new opportunities to improve the BBB-on-a-chip platform via multidisciplinary approaches are available. In this review, an overview of the NVU and OoC technology is provided, recent progress and applications of BBB-on-a-chip for personalized medicine and drug discovery are discussed, and current challenges and future directions are delineated.
Keywords: blood-brain barriers; disease modeling; drug discovery; organ-on-a-chips; personalized medicine.
© 2022 Wiley-VCH GmbH.
Conflict of interest statement
Conflict of Interest
The authors have no conflict of interest.
Figures
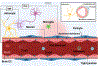
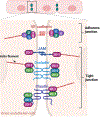
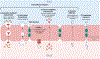
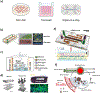
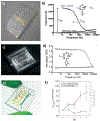
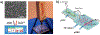
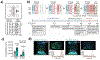
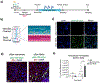
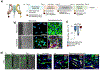
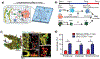
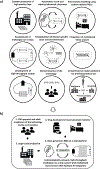
Similar articles
-
Blood-brain barrier (BBB)-on-a-chip: a promising breakthrough in brain disease research.Lab Chip. 2022 Sep 27;22(19):3579-3602. doi: 10.1039/d2lc00305h. Lab Chip. 2022. PMID: 36004771 Review.
-
Advanced Human BBB-on-a-Chip: A New Platform for Alzheimer's Disease Studies.Adv Healthc Mater. 2021 Aug;10(15):e2002285. doi: 10.1002/adhm.202002285. Epub 2021 Jun 2. Adv Healthc Mater. 2021. PMID: 34075728 Free PMC article. Review.
-
Generation of a Human iPSC-Based Blood-Brain Barrier Chip.J Vis Exp. 2020 Mar 2;(157). doi: 10.3791/60925. J Vis Exp. 2020. PMID: 32176199
-
Blood-brain barrier-on-a-chip: Microphysiological systems that capture the complexity of the blood-central nervous system interface.Exp Biol Med (Maywood). 2017 Nov;242(17):1669-1678. doi: 10.1177/1535370217694100. Epub 2017 Feb 14. Exp Biol Med (Maywood). 2017. PMID: 28195514 Free PMC article. Review.
-
In vitro modeling of the neurovascular unit: advances in the field.Fluids Barriers CNS. 2020 Mar 16;17(1):22. doi: 10.1186/s12987-020-00183-7. Fluids Barriers CNS. 2020. PMID: 32178700 Free PMC article. Review.
Cited by
-
Fallopian tube rheology regulates epithelial cell differentiation and function to enhance cilia formation and coordination.Nat Commun. 2024 Aug 28;15(1):7411. doi: 10.1038/s41467-024-51481-9. Nat Commun. 2024. PMID: 39198453 Free PMC article.
-
Current progress and challenges in the development of brain tissue models: How to grow up the changeable brain in vitro?J Tissue Eng. 2024 Mar 20;15:20417314241235527. doi: 10.1177/20417314241235527. eCollection 2024 Jan-Dec. J Tissue Eng. 2024. PMID: 38516227 Free PMC article. Review.
-
Anatomically and Physiologically Accurate Engineered Neurovascular Unit and Blood-Brain Barrier Model Using Microvessels Isolated from Postmortem Human Brain Tissue.bioRxiv [Preprint]. 2024 Sep 29:2024.09.26.615283. doi: 10.1101/2024.09.26.615283. bioRxiv. 2024. PMID: 39386654 Free PMC article. Preprint.
-
Customizable Microfluidic Devices: Progress, Constraints, and Future Advances.Curr Drug Deliv. 2024;21(10):1285-1299. doi: 10.2174/0115672018264064231017113813. Curr Drug Deliv. 2024. PMID: 39034714 Review.
-
Complex in vitro Model: A Transformative Model in Drug Development and Precision Medicine.Clin Transl Sci. 2023 Dec 7;17(2):e13695. doi: 10.1111/cts.13695. Online ahead of print. Clin Transl Sci. 2023. PMID: 38062923 Free PMC article. Review.
References
-
- Association A. s., Alzheimer’s & Dementia 2018, 14 (3), 367–429. DOI 10.1016/j.jalz.2018.02.001. - DOI
Publication types
MeSH terms
Grants and funding
LinkOut - more resources
Full Text Sources
Medical