Antimicrobial Proteins and Peptides in Avian Eggshell: Structural Diversity and Potential Roles in Biomineralization
- PMID: 35967448
- PMCID: PMC9363672
- DOI: 10.3389/fimmu.2022.946428
Antimicrobial Proteins and Peptides in Avian Eggshell: Structural Diversity and Potential Roles in Biomineralization
Abstract
The calcitic avian eggshell provides physical protection for the embryo during its development, but also regulates water and gaseous exchange, and is a calcium source for bone mineralization. The calcified eggshell has been extensively investigated in the chicken. It is characterized by an inventory of more than 900 matrix proteins. In addition to proteins involved in shell mineralization and regulation of its microstructure, the shell also contains numerous antimicrobial proteins and peptides (AMPPs) including lectin-like proteins, Bacterial Permeability Increasing/Lipopolysaccharide Binding Protein/PLUNC family proteins, defensins, antiproteases, and chelators, which contribute to the innate immune protection of the egg. In parallel, some of these proteins are thought to be crucial determinants of the eggshell texture and its resulting mechanical properties. During the progressive solubilization of the inner mineralized eggshell during embryonic development (to provide calcium to the embryo), some antimicrobials may be released simultaneously to reinforce egg defense and protect the egg from contamination by external pathogens, through a weakened eggshell. This review provides a comprehensive overview of the diversity of avian eggshell AMPPs, their three-dimensional structures and their mechanism of antimicrobial activity. The published chicken eggshell proteome databases are integrated for a comprehensive inventory of its AMPPs. Their biochemical features, potential dual function as antimicrobials and as regulators of eggshell biomineralization, and their phylogenetic evolution will be described and discussed with regard to their three-dimensional structural characteristics. Finally, the repertoire of chicken eggshell AMPPs are compared to orthologs identified in other avian and non-avian eggshells. This approach sheds light on the similarities and differences exhibited by AMPPs, depending on bird species, and leads to a better understanding of their sequential or dual role in biomineralization and innate immunity.
Keywords: 3D protein structure; antimicrobial peptides and proteins; avian egg; biomineralizing properties; calcite; eggshell.
Copyright © 2022 Moreau, Gautron, Hincke, Monget, Réhault-Godbert and Guyot.
Conflict of interest statement
The authors declare that the research was conducted in the absence of any commercial or financial relationships that could be construed as a potential conflict of interest.
Figures
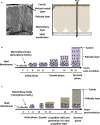
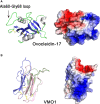
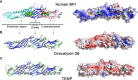
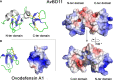
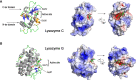
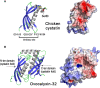
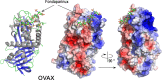
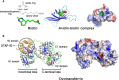
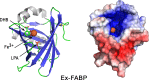
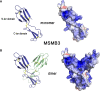
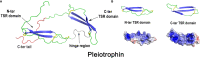
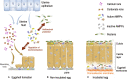
Similar articles
-
Guinea fowl eggshell quantitative proteomics yield new findings related to its unique structural characteristics and superior mechanical properties.J Proteomics. 2019 Oct 30;209:103511. doi: 10.1016/j.jprot.2019.103511. Epub 2019 Sep 5. J Proteomics. 2019. PMID: 31493547
-
Avian eggshell biomineralization: an update on its structure, mineralogy and protein tool kit.BMC Mol Cell Biol. 2021 Feb 12;22(1):11. doi: 10.1186/s12860-021-00350-0. BMC Mol Cell Biol. 2021. PMID: 33579194 Free PMC article. Review.
-
Novel identification of matrix proteins involved in calcitic biomineralization.J Proteomics. 2015 Feb 26;116:81-96. doi: 10.1016/j.jprot.2015.01.002. Epub 2015 Jan 10. J Proteomics. 2015. PMID: 25585129
-
Quantitative proteomics and bioinformatic analysis provide new insight into protein function during avian eggshell biomineralization.J Proteomics. 2015 Jan 15;113:178-93. doi: 10.1016/j.jprot.2014.09.024. Epub 2014 Oct 5. J Proteomics. 2015. PMID: 25284052
-
Protein constituents of the eggshell: eggshell-specific matrix proteins.Cell Mol Life Sci. 2009 Aug;66(16):2707-19. doi: 10.1007/s00018-009-0046-y. Epub 2009 May 19. Cell Mol Life Sci. 2009. PMID: 19452125 Free PMC article. Review.
References
Publication types
MeSH terms
Substances
LinkOut - more resources
Full Text Sources