Seipin concentrates distinct neutral lipids via interactions with their acyl chain carboxyl esters
- PMID: 35938957
- PMCID: PMC9365673
- DOI: 10.1083/jcb.202112068
Seipin concentrates distinct neutral lipids via interactions with their acyl chain carboxyl esters
Abstract
Lipid droplets (LDs) are essential for cellular lipid homeostasis by storing diverse neutral lipids (NLs), such as triacylglycerol (TAG), steryl esters (SE), and retinyl esters (RE). A proper assembly of TAG-containing LDs at the ER requires Seipin, a conserved protein often mutated in lipodystrophies. Here, we show that the yeast Seipin Sei1 and its partner Ldb16 also promote the storage of other NL in LDs. Importantly, this role of Sei1/Ldb16 is evolutionarily conserved as expression of human-Seipin restored normal SE-containing LDs in yeast Seipin mutants. As in the case of TAG, the formation of SE-containing LDs requires interactions between hydroxyl-residues in human Seipin or yeast Ldb16 with NL carboxyl esters. These findings provide a universal mechanism for Seipin-mediated LD formation and suggest a model for how Seipin distinguishes NLs from aliphatic phospholipid acyl chains in the center of the membrane bilayer.
© 2022 Renne et al.
Figures
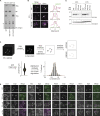
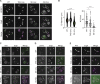
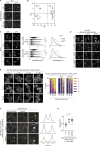
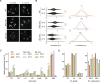
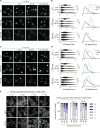
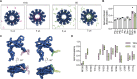
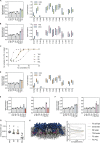
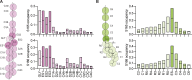
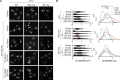
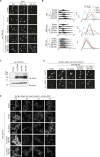
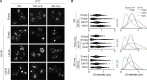
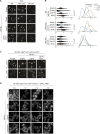
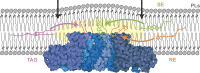
Similar articles
-
A unifying mechanism for seipin-mediated lipid droplet formation.FEBS Lett. 2024 May;598(10):1116-1126. doi: 10.1002/1873-3468.14825. Epub 2024 Feb 13. FEBS Lett. 2024. PMID: 38785192 Free PMC article. Review.
-
Mechanism of lipid droplet formation by the yeast Sei1/Ldb16 Seipin complex.Nat Commun. 2021 Oct 8;12(1):5892. doi: 10.1038/s41467-021-26162-6. Nat Commun. 2021. PMID: 34625558 Free PMC article.
-
Dissecting seipin function: the localized accumulation of phosphatidic acid at ER/LD junctions in the absence of seipin is suppressed by Sei1p(ΔNterm) only in combination with Ldb16p.BMC Cell Biol. 2015 Dec 4;16:29. doi: 10.1186/s12860-015-0075-3. BMC Cell Biol. 2015. PMID: 26637296 Free PMC article.
-
Control of lipid droplet size in budding yeast requires the collaboration between Fld1 and Ldb16.J Cell Sci. 2014 Mar 15;127(Pt 6):1214-28. doi: 10.1242/jcs.137737. Epub 2014 Jan 16. J Cell Sci. 2014. PMID: 24434579
-
SEIPIN: A Key Factor for Nuclear Lipid Droplet Generation and Lipid Homeostasis.Int J Mol Sci. 2020 Nov 2;21(21):8208. doi: 10.3390/ijms21218208. Int J Mol Sci. 2020. PMID: 33147895 Free PMC article. Review.
Cited by
-
Seipin deficiency-induced lipid dysregulation leads to hypomyelination-associated cognitive deficits via compromising oligodendrocyte precursor cell differentiation.Cell Death Dis. 2024 May 21;15(5):350. doi: 10.1038/s41419-024-06737-z. Cell Death Dis. 2024. PMID: 38773070 Free PMC article.
-
Cholesterol esters form supercooled lipid droplets whose nucleation is facilitated by triacylglycerols.Nat Commun. 2023 Feb 17;14(1):915. doi: 10.1038/s41467-023-36375-6. Nat Commun. 2023. PMID: 36807572 Free PMC article.
-
Seipin-still a mysterious protein?Front Cell Dev Biol. 2023 Feb 3;11:1112954. doi: 10.3389/fcell.2023.1112954. eCollection 2023. Front Cell Dev Biol. 2023. PMID: 36819093 Free PMC article. Review.
-
A unifying mechanism for seipin-mediated lipid droplet formation.FEBS Lett. 2024 May;598(10):1116-1126. doi: 10.1002/1873-3468.14825. Epub 2024 Feb 13. FEBS Lett. 2024. PMID: 38785192 Free PMC article. Review.
-
Hairpin protein partitioning from the ER to lipid droplets involves major structural rearrangements.Nat Commun. 2024 May 27;15(1):4504. doi: 10.1038/s41467-024-48843-8. Nat Commun. 2024. PMID: 38802378 Free PMC article.
References
-
- Abraham, M.J., Murtola T., Schulz R., Páll S., Smith J.C., Hess B., and Lindahl E.. 2015. GROMACS: High performance molecular simulations through multi-level parallelism from laptops to supercomputers. SoftwareX. 1–2:19–25. 10.1016/j.softx.2015.06.001 - DOI
Publication types
MeSH terms
Substances
Grants and funding
- BB/S003339/1/BB_/Biotechnology and Biological Sciences Research Council/United Kingdom
- BB/R018375/1/BB_/Biotechnology and Biological Sciences Research Council/United Kingdom
- 202642/Z/16/Z/WT_/Wellcome Trust/United Kingdom
- BB/R002517/1/BB_/Biotechnology and Biological Sciences Research Council/United Kingdom
- BB/P01948X/1/BB_/Biotechnology and Biological Sciences Research Council/United Kingdom
LinkOut - more resources
Full Text Sources
Molecular Biology Databases
Research Materials