Landscape of lipidomic metabolites in gut-liver axis of Sprague-Dawley rats after oral exposure to titanium dioxide nanoparticles
- PMID: 35922847
- PMCID: PMC9351087
- DOI: 10.1186/s12989-022-00484-9
Landscape of lipidomic metabolites in gut-liver axis of Sprague-Dawley rats after oral exposure to titanium dioxide nanoparticles
Abstract
Background: The application of titanium dioxide nanoparticles (TiO2 NPs) as food additives poses a risk of oral exposure that may lead to adverse health effects. Even though the substantial evidence supported liver as the target organ of TiO2 NPs via oral exposure, the mechanism of liver toxicity remains largely unknown. Since the liver is a key organ for lipid metabolism, this study focused on the landscape of lipidomic metabolites in gut-liver axis of Sprague Dawley (SD) rats exposed to TiO2 NPs at 0, 2, 10, 50 mg/kg body weight per day for 90 days.
Results: TiO2 NPs (50 mg/kg) caused slight hepatotoxicity and changed lipidomic signatures of main organs or systems in the gut-liver axis including liver, serum and gut. The cluster profile from the above biological samples all pointed to the same key metabolic pathway and metabolites, which was glycerophospholipid metabolism and Phosphatidylcholines (PCs), respectively. In addition, absolute quantitative lipidomics verified the changes of three PCs concentrations, including PC (16:0/20:1), PC (18:0/18:0) and PC (18:2/20:2) in the serum samples after treatment of TiO2 NPs (50 mg/kg). The contents of malondialdehyde (MDA) in serum and liver increased significantly, which were positively correlated with most differential lipophilic metabolites.
Conclusions: The gut was presumed to be the original site of oxidative stress and disorder of lipid metabolism, which resulted in hepatotoxicity through the gut-liver axis. Lipid peroxidation may be the initial step of lipid metabolism disorder induced by TiO2 NPs. Most nanomaterials (NMs) have oxidation induction and antibacterial properties, so the toxic pathway revealed in the present study may be primary and universal.
Keywords: Gut-liver axis; Lipid peroxidation; Lipidomics; Nanotoxicity; Titanium dioxide nanoparticles.
© 2022. The Author(s).
Conflict of interest statement
The authors declare that they have no competing financial interests.
Figures
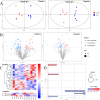
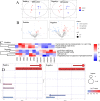
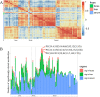
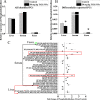
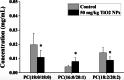
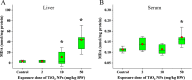
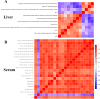
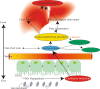
Similar articles
-
Titanium dioxide nanoparticles: revealing the mechanisms underlying hepatotoxicity and effects in the gut microbiota.Arch Toxicol. 2023 Aug;97(8):2051-2067. doi: 10.1007/s00204-023-03536-x. Epub 2023 Jun 22. Arch Toxicol. 2023. PMID: 37344693 Review.
-
Hepatotoxicity and the role of the gut-liver axis in rats after oral administration of titanium dioxide nanoparticles.Part Fibre Toxicol. 2019 Dec 27;16(1):48. doi: 10.1186/s12989-019-0332-2. Part Fibre Toxicol. 2019. PMID: 31881974 Free PMC article.
-
Effect of oral exposure to titanium dioxide nanoparticles on lipid metabolism in Sprague-Dawley rats.Nanoscale. 2020 Mar 12;12(10):5973-5986. doi: 10.1039/c9nr10947a. Nanoscale. 2020. PMID: 32108206
-
Effects of oral exposure to titanium dioxide nanoparticles on gut microbiota and gut-associated metabolism in vivo.Nanoscale. 2019 Nov 28;11(46):22398-22412. doi: 10.1039/c9nr07580a. Nanoscale. 2019. PMID: 31738363
-
Dysregulation along the gut microbiota-immune system axis after oral exposure to titanium dioxide nanoparticles: A possible environmental factor promoting obesity-related metabolic disorders.Environ Pollut. 2023 Aug 1;330:121795. doi: 10.1016/j.envpol.2023.121795. Epub 2023 May 13. Environ Pollut. 2023. PMID: 37187281 Review.
Cited by
-
Molecular mechanism of nanomaterials induced liver injury: A review.World J Hepatol. 2024 Apr 27;16(4):566-600. doi: 10.4254/wjh.v16.i4.566. World J Hepatol. 2024. PMID: 38689743 Free PMC article. Review.
-
Titanium dioxide nanoparticles: revealing the mechanisms underlying hepatotoxicity and effects in the gut microbiota.Arch Toxicol. 2023 Aug;97(8):2051-2067. doi: 10.1007/s00204-023-03536-x. Epub 2023 Jun 22. Arch Toxicol. 2023. PMID: 37344693 Review.
-
Alleviative Effect of Lactoferrin Interventions Against the Hepatotoxicity Induced by Titanium Dioxide Nanoparticles.Biol Trace Elem Res. 2024 Feb;202(2):624-642. doi: 10.1007/s12011-023-03702-3. Epub 2023 May 16. Biol Trace Elem Res. 2024. PMID: 37191759
-
Medical and Dental Applications of Titania Nanoparticles: An Overview.Nanomaterials (Basel). 2022 Oct 19;12(20):3670. doi: 10.3390/nano12203670. Nanomaterials (Basel). 2022. PMID: 36296859 Free PMC article. Review.
References
-
- Keller AA, McFerran S, Lazareva A, Suh S. Global life cycle releases of engineered nanomaterials. J Nanopart Res. 2013;15(6):1692. doi: 10.1007/s11051-013-1692-4. - DOI
-
- Piccinno F, Gottschalk F, Seeger S, Nowack B. Industrial production quantities and uses of ten engineered nanomaterials in Europe and the world. J Nanopart Res. 2012;14(9):1109. doi: 10.1007/s11051-012-1109-9. - DOI
Publication types
MeSH terms
Substances
LinkOut - more resources
Full Text Sources
Medical