Pathogenic bacteria remodel central metabolic enzyme to build a cyclopropanol warhead
- PMID: 35906404
- PMCID: PMC9359912
- DOI: 10.1038/s41557-022-01005-z
Pathogenic bacteria remodel central metabolic enzyme to build a cyclopropanol warhead
Abstract
Bacteria of the Burkholderia pseudomallei (BP) group pose a global health threat, causing the infectious diseases melioidosis, a common cause of pneumonia and sepsis, and glanders, a contagious zoonosis. A trait of BP bacteria is a conserved gene cluster coding for the biosynthesis of polyketides (malleicyprols) with a reactive cyclopropanol unit that is critical for virulence. Enzymes building this warhead represent ideal targets for antivirulence strategies but the biochemical basis of cyclopropanol formation is unknown. Here we describe the formation of the malleicyprol warhead. We show that BurG, an unusual NAD+-dependent member of the ketol-acid reductoisomerase family, constructs the strained cyclopropanol ring. Biochemical assays and a suite of eight crystal structures of native and mutated BurG with bound analogues and inhibitors provide snapshots of each step of the complex reaction mechanism, involving a concealed oxidoreduction and a C-S bond cleavage. Our findings illustrate a remarkable case of neofunctionalisation, where a biocatalyst from central metabolism has been evolutionarily repurposed for warhead production in pathogens.
© 2022. The Author(s).
Conflict of interest statement
The authors declare no competing interests.
Figures
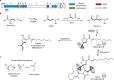
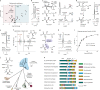
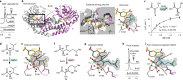
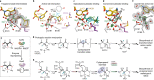
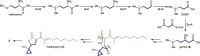
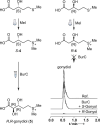
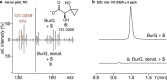
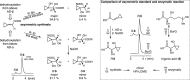
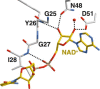
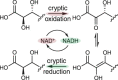
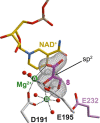
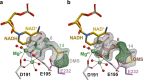
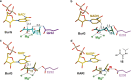
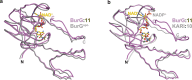
Similar articles
-
Molecular insights into Burkholderia pseudomallei and Burkholderia mallei pathogenesis.Annu Rev Microbiol. 2010;64:495-517. doi: 10.1146/annurev.micro.112408.134030. Annu Rev Microbiol. 2010. PMID: 20528691 Review.
-
Cyclopropanol Warhead in Malleicyprol Confers Virulence of Human- and Animal-Pathogenic Burkholderia Species.Angew Chem Int Ed Engl. 2019 Oct 1;58(40):14129-14133. doi: 10.1002/anie.201907324. Epub 2019 Aug 27. Angew Chem Int Ed Engl. 2019. PMID: 31353766 Free PMC article.
-
Development of hydrolysis probe-based real-time PCR for identification of virulent gene targets of Burkholderia pseudomallei and B. mallei--a retrospective study on archival cases of service members with melioidosis and glanders.Mil Med. 2012 Feb;177(2):216-21. doi: 10.7205/milmed-d-11-00232. Mil Med. 2012. PMID: 22360070
-
Use of a safe, reproducible, and rapid aerosol delivery method to study infection by Burkholderia pseudomallei and Burkholderia mallei in mice.PLoS One. 2013 Oct 2;8(10):e76804. doi: 10.1371/journal.pone.0076804. eCollection 2013. PLoS One. 2013. PMID: 24098563 Free PMC article.
-
PCR-based Methodologies Used to Detect and Differentiate the Burkholderia pseudomallei complex: B. pseudomallei, B. mallei, and B. thailandensis.Curr Issues Mol Biol. 2014;16:23-54. Epub 2013 Aug 22. Curr Issues Mol Biol. 2014. PMID: 23969318 Review.
Cited by
-
Post-Cyclization Skeletal Rearrangements in Plant Triterpenoid Biosynthesis by a Pair of Branchpoint Isomerases.J Am Chem Soc. 2023 Mar 8;145(9):5083-5091. doi: 10.1021/jacs.2c10838. Epub 2023 Feb 23. J Am Chem Soc. 2023. PMID: 36821810 Free PMC article.
-
An NmrA-like enzyme-catalysed redox-mediated Diels-Alder cycloaddition with anti-selectivity.Nat Chem. 2023 Apr;15(4):526-534. doi: 10.1038/s41557-022-01117-6. Epub 2023 Jan 12. Nat Chem. 2023. PMID: 36635598 Free PMC article.
-
Bioinformatics-guided discovery of biaryl-linked lasso peptides.Chem Sci. 2023 Oct 30;14(45):13176-13183. doi: 10.1039/d3sc02380j. eCollection 2023 Nov 22. Chem Sci. 2023. PMID: 38023510 Free PMC article.
-
An intramodular thioesterase domain catalyses chain release in the biosynthesis of a cytotoxic virulence factor.RSC Chem Biol. 2022 Jul 25;3(9):1121-1128. doi: 10.1039/d2cb00121g. eCollection 2022 Aug 31. RSC Chem Biol. 2022. PMID: 36128506 Free PMC article.
-
Bioinformatics-Guided Discovery of Biaryl-Tailored Lasso Peptides.bioRxiv [Preprint]. 2023 Mar 6:2023.03.06.531328. doi: 10.1101/2023.03.06.531328. bioRxiv. 2023. Update in: Chem Sci. 2023 Oct 30;14(45):13176-13183. doi: 10.1039/d3sc02380j. PMID: 36945544 Free PMC article. Updated. Preprint.
References
-
- Galyov EE, Brett PJ, DeShazer D. Molecular insights into Burkholderia pseudomallei and Burkholderia mallei pathogenesis. Annu. Rev. Microbiol. 2010;64:495–517. - PubMed
-
- Franke J, Ishida K, Hertweck C. Evolution of siderophore pathways in human pathogenic bacteria. J. Am. Chem. Soc. 2014;136:5599–5602. - PubMed
-
- Franke J, Ishida K, Hertweck C. Plasticity of the malleobactin pathway and its impact on siderophore action in human pathogenic bacteria. Chem. Eur. J. 2015;21:8010–8014. - PubMed
-
- Franke J, Ishida K, Ishida-Ito M, Hertweck C. Nitro versus hydroxamate in siderophores of pathogenic bacteria: effect of missing hydroxylamine protection in malleobactin biosynthesis. Angew. Chem. Int. Ed. 2013;52:8271–8275. - PubMed
Publication types
MeSH terms
Substances
LinkOut - more resources
Full Text Sources
Other Literature Sources