ATP-competitive inhibitors modulate the substrate binding cooperativity of a kinase by altering its conformational entropy
- PMID: 35905186
- PMCID: PMC9337769
- DOI: 10.1126/sciadv.abo0696
ATP-competitive inhibitors modulate the substrate binding cooperativity of a kinase by altering its conformational entropy
Abstract
ATP-competitive inhibitors are currently the largest class of clinically approved drugs for protein kinases. By targeting the ATP-binding pocket, these compounds block the catalytic activity, preventing substrate phosphorylation. A problem with these drugs, however, is that inhibited kinases may still recognize and bind downstream substrates, acting as scaffolds or binding hubs for signaling partners. Here, using protein kinase A as a model system, we show that chemically different ATP-competitive inhibitors modulate the substrate binding cooperativity by tuning the conformational entropy of the kinase and shifting the populations of its conformationally excited states. Since we found that binding cooperativity and conformational entropy of the enzyme are correlated, we propose a new paradigm for the discovery of ATP-competitive inhibitors, which is based on their ability to modulate the allosteric coupling between nucleotide and substrate-binding sites.
Figures
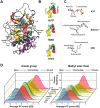
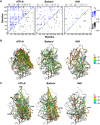
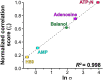
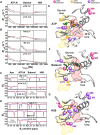
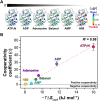
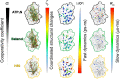
Similar articles
-
The αC-β4 loop controls the allosteric cooperativity between nucleotide and substrate in the catalytic subunit of protein kinase A.bioRxiv [Preprint]. 2023 Sep 15:2023.09.12.557419. doi: 10.1101/2023.09.12.557419. bioRxiv. 2023. Update in: Elife. 2024 Jun 24;12:RP91506. doi: 10.7554/eLife.91506. PMID: 37745542 Free PMC article. Updated. Preprint.
-
The αC-β4 loop controls the allosteric cooperativity between nucleotide and substrate in the catalytic subunit of protein kinase A.Elife. 2024 Jun 24;12:RP91506. doi: 10.7554/eLife.91506. Elife. 2024. PMID: 38913408 Free PMC article.
-
Allosteric Modulation of JNK Docking Site Interactions with ATP-Competitive Inhibitors.Biochemistry. 2018 Oct 9;57(40):5897-5909. doi: 10.1021/acs.biochem.8b00776. Epub 2018 Sep 25. Biochemistry. 2018. PMID: 30211540 Free PMC article.
-
An NMR portrait of functional and dysfunctional allosteric cooperativity in cAMP-dependent protein kinase A.FEBS Lett. 2023 Apr;597(8):1055-1072. doi: 10.1002/1873-3468.14610. Epub 2023 Mar 26. FEBS Lett. 2023. PMID: 36892429 Free PMC article. Review.
-
A historical overview of protein kinases and their targeted small molecule inhibitors.Pharmacol Res. 2015 Oct;100:1-23. doi: 10.1016/j.phrs.2015.07.010. Epub 2015 Jul 21. Pharmacol Res. 2015. PMID: 26207888 Review.
Cited by
-
Programming conformational cooperativity to regulate allosteric protein-oligonucleotide signal transduction.Nat Commun. 2023 Aug 14;14(1):4898. doi: 10.1038/s41467-023-40589-z. Nat Commun. 2023. PMID: 37580346 Free PMC article.
-
Conformation Selection by ATP-competitive Inhibitors and Allosteric Communication in ERK2.bioRxiv [Preprint]. 2023 Nov 6:2023.09.12.557258. doi: 10.1101/2023.09.12.557258. bioRxiv. 2023. Update in: Elife. 2024 Mar 27;12:RP91507. doi: 10.7554/eLife.91507. PMID: 37745518 Free PMC article. Updated. Preprint.
-
CDK2 and CDK4: Cell Cycle Functions Evolve Distinct, Catalysis-Competent Conformations, Offering Drug Targets.JACS Au. 2024 May 14;4(5):1911-1927. doi: 10.1021/jacsau.4c00138. eCollection 2024 May 27. JACS Au. 2024. PMID: 38818077 Free PMC article.
-
Allo-targeting of the kinase domain: Insights from in silico studies and comparison with experiments.Curr Opin Struct Biol. 2024 Feb;84:102770. doi: 10.1016/j.sbi.2023.102770. Epub 2024 Jan 11. Curr Opin Struct Biol. 2024. PMID: 38211377 Free PMC article. Review.
-
AI-designed NMR spectroscopy RF pulses for fast acquisition at high and ultra-high magnetic fields.Nat Commun. 2023 Jul 12;14(1):4144. doi: 10.1038/s41467-023-39581-4. Nat Commun. 2023. PMID: 37438347 Free PMC article.
References
-
- Manning G., Whyte D. B., Martinez R., Hunter T., Sudarsanam S., The protein kinase complement of the human genome. Science 298, 1912–1934 (2002). - PubMed
-
- Q. Wang, J. A. Zorn, J. Kuriyan, in Methods in Enzymology, K. M. Shokat, Ed. (Academic Press, 2014), vol. 548, pp. 23–67. - PubMed
-
- Dar A. C., Shokat K. M., The evolution of protein kinase inhibitors from antagonists to agonists of cellular signaling. Annu. Rev. Biochem. 80, 769–795 (2011). - PubMed
-
- Tong M., Seeliger M. A., Targeting conformational plasticity of protein kinases. ACS Chem. Biol. 10, 190–200 (2015). - PubMed
-
- Boudeau J., Miranda-Saavedra D., Barton G. J., Alessi D. R., Emerging roles of pseudokinases. Trends Cell Biol. 16, 443–452 (2006). - PubMed