Inimitable Impacts of Ceramides on Lipid Rafts Formed in Artificial and Natural Cell Membranes
- PMID: 35893445
- PMCID: PMC9330320
- DOI: 10.3390/membranes12080727
Inimitable Impacts of Ceramides on Lipid Rafts Formed in Artificial and Natural Cell Membranes
Abstract
Ceramide is the simplest precursor of sphingolipids and is involved in a variety of biological functions ranging from apoptosis to the immune responses. Although ceramide is a minor constituent of plasma membranes, it drastically increases upon cellular stimulation. However, the mechanistic link between ceramide generation and signal transduction remains unknown. To address this issue, the effect of ceramide on phospholipid membranes has been examined in numerous studies. One of the most remarkable findings of these studies is that ceramide induces the coalescence of membrane domains termed lipid rafts. Thus, it has been hypothesised that ceramide exerts its biological activity through the structural alteration of lipid rafts. In the present article, we first discuss the characteristic hydrogen bond functionality of ceramides. Then, we showed the impact of ceramide on the structures of artificial and cell membranes, including the coalescence of the pre-existing lipid raft into a large patch called a signal platform. Moreover, we proposed a possible structure of the signal platform, in which sphingomyelin/cholesterol-rich and sphingomyelin/ceramide-rich domains coexist. This structure is considered to be beneficial because membrane proteins and their inhibitors are separately compartmentalised in those domains. Considering the fact that ceramide/cholesterol content regulates the miscibility of those two domains in model membranes, the association and dissociation of membrane proteins and their inhibitors might be controlled by the contents of ceramide and cholesterol in the signal platform.
Keywords: apoptosis; lipid membranes; lipid rafts; phase separation; signal platforms; transmembrane signalling.
Conflict of interest statement
The authors declare no conflict of interest.
Figures
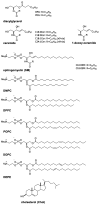
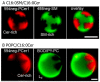
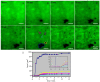
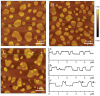
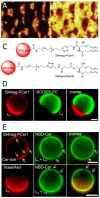
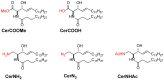
Similar articles
-
Ceramide selectively displaces cholesterol from ordered lipid domains (rafts): implications for lipid raft structure and function.J Biol Chem. 2004 Mar 12;279(11):9997-10004. doi: 10.1074/jbc.M309992200. Epub 2003 Dec 29. J Biol Chem. 2004. PMID: 14699154
-
Effect of ceramide N-acyl chain and polar headgroup structure on the properties of ordered lipid domains (lipid rafts).Biochim Biophys Acta. 2007 Sep;1768(9):2205-12. doi: 10.1016/j.bbamem.2007.05.007. Epub 2007 May 13. Biochim Biophys Acta. 2007. PMID: 17574203 Free PMC article.
-
Cholesterol-rich fluid membranes solubilize ceramide domains: implications for the structure and dynamics of mammalian intracellular and plasma membranes.J Biol Chem. 2009 Aug 21;284(34):22978-87. doi: 10.1074/jbc.M109.026567. Epub 2009 Jun 11. J Biol Chem. 2009. PMID: 19520848 Free PMC article.
-
Sphingolipid signalling domains floating on rafts or buried in caves?Cell Signal. 2000 Feb;12(2):81-90. doi: 10.1016/s0898-6568(99)00072-8. Cell Signal. 2000. PMID: 10679576 Review.
-
Ceramide: a simple sphingolipid with unique biophysical properties.Prog Lipid Res. 2014 Apr;54:53-67. doi: 10.1016/j.plipres.2014.01.004. Epub 2014 Feb 7. Prog Lipid Res. 2014. PMID: 24513486 Review.
Cited by
-
Quantitative Lipid Profiling Reveals Major Differences between Liver Organoids with Normal Pi*M and Deficient Pi*Z Variants of Alpha-1-antitrypsin.Int J Mol Sci. 2023 Aug 5;24(15):12472. doi: 10.3390/ijms241512472. Int J Mol Sci. 2023. PMID: 37569847 Free PMC article.
-
Next-level multiomics helps define new critical pathways in developmental hematopoiesis.Hemasphere. 2024 Feb 1;8(2):e42. doi: 10.1002/hem3.42. eCollection 2024 Feb. Hemasphere. 2024. PMID: 38435421 Free PMC article. No abstract available.
-
Salmonella Type III Secretion Effector SrfJ: A Glucosylceramidase Affecting the Lipidome and the Transcriptome of Mammalian Host Cells.Int J Mol Sci. 2023 May 7;24(9):8403. doi: 10.3390/ijms24098403. Int J Mol Sci. 2023. PMID: 37176110 Free PMC article.
-
Ceramides are fuel gauges on the drive to cardiometabolic disease.Physiol Rev. 2024 Jul 1;104(3):1061-1119. doi: 10.1152/physrev.00008.2023. Epub 2024 Feb 1. Physiol Rev. 2024. PMID: 38300524 Review.
-
Zymosan-Induced Murine Peritonitis Is Associated with an Increased Sphingolipid Synthesis without Changing the Long to Very Long Chain Ceramide Ratio.Int J Mol Sci. 2023 Feb 1;24(3):2773. doi: 10.3390/ijms24032773. Int J Mol Sci. 2023. PMID: 36769096 Free PMC article.
References
-
- Shabbir M.A., Mehak F., Khan Z.M., Ahmad W., Khan M.R., Zia S., Rahaman A., Aadil R.M. Interplay between ceramides and phytonutrients: New insights in metabolic syndrome. Trends Food Sci. Technol. 2021;111:483–494. doi: 10.1016/j.tifs.2021.03.010. - DOI
Publication types
Grants and funding
LinkOut - more resources
Full Text Sources