Mutation of foxl1 Results in Reduced Cartilage Markers in a Zebrafish Model of Otosclerosis
- PMID: 35885890
- PMCID: PMC9319681
- DOI: 10.3390/genes13071107
Mutation of foxl1 Results in Reduced Cartilage Markers in a Zebrafish Model of Otosclerosis
Abstract
Bone diseases such as otosclerosis (conductive hearing loss) and osteoporosis (low bone mineral density) can result from the abnormal expression of genes that regulate cartilage and bone development. The forkhead box transcription factor FOXL1 has been identified as the causative gene in a family with autosomal dominant otosclerosis and has been reported as a candidate gene in GWAS meta-analyses for osteoporosis. This potentially indicates a novel role for foxl1 in chondrogenesis, osteogenesis, and bone remodelling. We created a foxl1 mutant zebrafish strain as a model for otosclerosis and osteoporosis and examined jaw bones that are homologous to the mammalian middle ear bones, and mineralization of the axial skeleton. We demonstrate that foxl1 regulates the expression of collagen genes such as collagen type 1 alpha 1a and collagen type 11 alpha 2, and results in a delay in jawbone mineralization, while the axial skeleton remains unchanged. foxl1 may also act with other forkhead genes such as foxc1a, as loss of foxl1 in a foxc1a mutant background increases the severity of jaw calcification phenotypes when compared to each mutant alone. Our zebrafish model demonstrates atypical cartilage formation and mineralization in the zebrafish craniofacial skeleton in foxl1 mutants and demonstrates that aberrant collagen expression may underlie the development of otosclerosis.
Keywords: bone mineral density; collagen; foxc1; foxl1; iron binding; osteoporosis; otosclerosis; zebrafish.
Conflict of interest statement
The authors declare no conflict of interest.
Figures
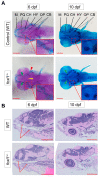
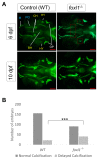
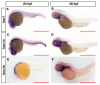
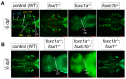
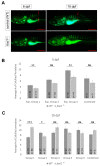
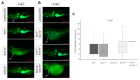
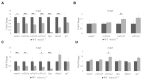
Similar articles
-
A pathogenic deletion in Forkhead Box L1 (FOXL1) identifies the first otosclerosis (OTSC) gene.Hum Genet. 2022 Apr;141(3-4):965-979. doi: 10.1007/s00439-021-02381-1. Epub 2021 Oct 11. Hum Genet. 2022. PMID: 34633540 Free PMC article.
-
Lrp5 Mutant and Crispant Zebrafish Faithfully Model Human Osteoporosis, Establishing the Zebrafish as a Platform for CRISPR-Based Functional Screening of Osteoporosis Candidate Genes.J Bone Miner Res. 2021 Sep;36(9):1749-1764. doi: 10.1002/jbmr.4327. Epub 2021 May 19. J Bone Miner Res. 2021. PMID: 33957005
-
Disruption of foxc1 genes in zebrafish results in dosage-dependent phenotypes overlapping Axenfeld-Rieger syndrome.Hum Mol Genet. 2020 Sep 29;29(16):2723-2735. doi: 10.1093/hmg/ddaa163. Hum Mol Genet. 2020. PMID: 32720677 Free PMC article.
-
Genetics of otosclerosis.Otol Neurotol. 2009 Dec;30(8):1021-32. doi: 10.1097/MAO.0b013e3181a86509. Otol Neurotol. 2009. PMID: 19546831 Review.
-
Molecular biology of otosclerosis.Adv Otorhinolaryngol. 2007;65:68-74. doi: 10.1159/000098674. Adv Otorhinolaryngol. 2007. PMID: 17245026 Review.
Cited by
-
Zebrafish as a Model for Osteoporosis: Functional Validations of Genome-Wide Association Studies.Curr Osteoporos Rep. 2023 Dec;21(6):650-659. doi: 10.1007/s11914-023-00831-5. Epub 2023 Nov 16. Curr Osteoporos Rep. 2023. PMID: 37971665 Review.
-
SMARCA4 mutation causes human otosclerosis and a similar phenotype in mice.J Med Genet. 2024 Jan 19;61(2):117-124. doi: 10.1136/jmg-2023-109264. J Med Genet. 2024. PMID: 37399313 Free PMC article.
References
-
- Javidan Y., Schilling T.F. Methods in Cell Biology. Volume 76. Elsevier; Amsterdam, The Netherlands: 2004. Development of Cartilage and Bone; pp. 415–436. - PubMed
Publication types
MeSH terms
Substances
Grants and funding
LinkOut - more resources
Full Text Sources
Medical
Molecular Biology Databases