Light Intensity- and Spectrum-Dependent Redox Regulation of Plant Metabolism
- PMID: 35883801
- PMCID: PMC9312225
- DOI: 10.3390/antiox11071311
Light Intensity- and Spectrum-Dependent Redox Regulation of Plant Metabolism
Abstract
Both light intensity and spectrum (280-800 nm) affect photosynthesis and, consequently, the formation of reactive oxygen species (ROS) during photosynthetic electron transport. ROS, together with antioxidants, determine the redox environment in tissues and cells, which in turn has a major role in the adjustment of metabolism to changes in environmental conditions. This process is very important since there are great spatial (latitude, altitude) and temporal (daily, seasonal) changes in light conditions which are accompanied by fluctuations in temperature, water supply, and biotic stresses. The blue and red spectral regimens are decisive in the regulation of metabolism because of the absorption maximums of chlorophylls and the sensitivity of photoreceptors. Based on recent publications, photoreceptor-controlled transcription factors such as ELONGATED HYPOCOTYL5 (HY5) and changes in the cellular redox environment may have a major role in the coordinated fine-tuning of metabolic processes during changes in light conditions. This review gives an overview of the current knowledge of the light-associated redox control of basic metabolic pathways (carbon, nitrogen, amino acid, sulphur, lipid, and nucleic acid metabolism), secondary metabolism (terpenoids, flavonoids, and alkaloids), and related molecular mechanisms. Light condition-related reprogramming of metabolism is the basis for proper growth and development of plants; therefore, its better understanding can contribute to more efficient crop production in the future.
Keywords: antioxidants; blue light; carbon assimilation; elongated hypocotyl5; far-red light; light intensity; lipid metabolism; nitrogen assimilation; photoreceptors; reactive oxygen species; red light; secondary metabolism; sulphur assimilation.
Conflict of interest statement
The authors declare no conflict of interest.
Figures
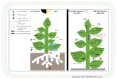
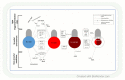
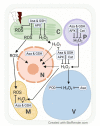
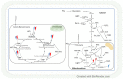
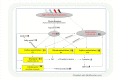
Similar articles
-
Light-Dependent Regulatory Interactions between the Redox System and miRNAs and Their Biochemical and Physiological Effects in Plants.Int J Mol Sci. 2023 May 5;24(9):8323. doi: 10.3390/ijms24098323. Int J Mol Sci. 2023. PMID: 37176028 Free PMC article. Review.
-
Far-Red Light Coordinates the Diurnal Changes in the Transcripts Related to Nitrate Reduction, Glutathione Metabolism and Antioxidant Enzymes in Barley.Int J Mol Sci. 2022 Jul 5;23(13):7479. doi: 10.3390/ijms23137479. Int J Mol Sci. 2022. PMID: 35806480 Free PMC article.
-
Overview of the physiological ecology of carbon metabolism in seagrasses.J Exp Mar Biol Ecol. 2000 Jul 30;250(1-2):169-205. doi: 10.1016/s0022-0981(00)00196-9. J Exp Mar Biol Ecol. 2000. PMID: 10969168
-
Impact of energy limitations on function and resilience in long-wavelength Photosystem II.Elife. 2022 Jul 19;11:e79890. doi: 10.7554/eLife.79890. Elife. 2022. PMID: 35852834 Free PMC article.
-
[Research advance in nitrogen metabolism of plant and its environmental regulation].Ying Yong Sheng Tai Xue Bao. 2004 Mar;15(3):511-6. Ying Yong Sheng Tai Xue Bao. 2004. PMID: 15228008 Review. Chinese.
Cited by
-
Management of Secondary Metabolite Synthesis and Biomass in Basil (Ocimum basilicum L.) Microgreens Using Different Continuous-Spectrum LED Lights.Plants (Basel). 2024 May 17;13(10):1394. doi: 10.3390/plants13101394. Plants (Basel). 2024. PMID: 38794463 Free PMC article.
-
Investigation of Arabidopsis root skototropism with different distance settings.Plant Signal Behav. 2024 Dec 31;19(1):2348917. doi: 10.1080/15592324.2024.2348917. Epub 2024 May 5. Plant Signal Behav. 2024. PMID: 38704856 Free PMC article.
-
Varying levels of natural light intensity affect the phyto-biochemical compounds, antioxidant indices and genes involved in the monoterpene biosynthetic pathway of Origanum majorana L.BMC Plant Biol. 2024 Oct 28;24(1):1018. doi: 10.1186/s12870-024-05739-5. BMC Plant Biol. 2024. PMID: 39465361 Free PMC article.
-
Potential Role of Phytochromes A and B and Cryptochrome 1 in the Adaptation of Solanum lycopersicum to UV-B Radiation.Int J Mol Sci. 2023 Aug 24;24(17):13142. doi: 10.3390/ijms241713142. Int J Mol Sci. 2023. PMID: 37685948 Free PMC article.
-
Light-Dependent Regulatory Interactions between the Redox System and miRNAs and Their Biochemical and Physiological Effects in Plants.Int J Mol Sci. 2023 May 5;24(9):8323. doi: 10.3390/ijms24098323. Int J Mol Sci. 2023. PMID: 37176028 Free PMC article. Review.
References
-
- Gröbner J., Albold A., Blumthaler M., Cabot T., De la Casiniere A., Lenoble J., Martin T., Masserot D., Müller M., Philipona R., et al. Variability of spectral solar ultraviolet irradiance in an Alpine environment. J. Geophys. Res. Atmos. 2000;105:26991–27003. doi: 10.1029/2000JD900395. - DOI
-
- Kotilainen T., Aphalo P.J., Brelsford C.C., Böök H., Devraj S., Heikkilä A., Hernández R., Kylling A., Lindfors A.V., Robson T.M. Patterns in the spectral composition of sunlight and biologically meaningful spectral photon ratios as affected by atmospheric factors. Agric. For. Meteorol. 2020;291:108041. doi: 10.1016/j.agrformet.2020.108041. - DOI
-
- Roro A.G., Terfa M.T., Solhaug K.A., Tsegaye A., Olsen J.E., Torre S. The impact of UV radiation at high altitudes close to the equator on morphology and productivity of pea (Pisum sativum) in different seasons. S. Afr. J. Bot. 2016;106:119–128. doi: 10.1016/j.sajb.2016.05.011. - DOI
-
- Holmes M.G., Smith H. The Function of Phytochrome in the Natural Environment—I. Characterization of Daylight for Studies in Photomorphogenesis and Photoperiodism. Photochem. Photobiol. 1977;25:533–538. doi: 10.1111/j.1751-1097.1977.tb09124.x. - DOI
Publication types
Grants and funding
LinkOut - more resources
Full Text Sources