Altered tRNA dynamics during translocation on slippery mRNA as determinant of spontaneous ribosome frameshifting
- PMID: 35869111
- PMCID: PMC9307594
- DOI: 10.1038/s41467-022-31852-w
Altered tRNA dynamics during translocation on slippery mRNA as determinant of spontaneous ribosome frameshifting
Abstract
When reading consecutive mRNA codons, ribosomes move by exactly one triplet at a time to synthesize a correct protein. Some mRNA tracks, called slippery sequences, are prone to ribosomal frameshifting, because the same tRNA can read both 0- and -1-frame codon. Using smFRET we show that during EF-G-catalyzed translocation on slippery sequences a fraction of ribosomes spontaneously switches from rapid, accurate translation to a slow, frameshifting-prone translocation mode where the movements of peptidyl- and deacylated tRNA become uncoupled. While deacylated tRNA translocates rapidly, pept-tRNA continues to fluctuate between chimeric and posttranslocation states, which slows down the re-locking of the small ribosomal subunit head domain. After rapid release of deacylated tRNA, pept-tRNA gains unconstrained access to the -1-frame triplet, resulting in slippage followed by recruitment of the -1-frame aa-tRNA into the A site. Our data show how altered choreography of tRNA and ribosome movements reduces the translation fidelity of ribosomes translocating in a slow mode.
© 2022. The Author(s).
Conflict of interest statement
The authors declare no competing interests.
Figures
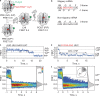
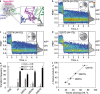
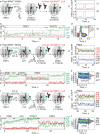
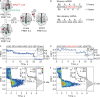
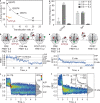
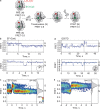
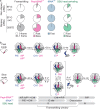
Similar articles
-
Structural basis for +1 ribosomal frameshifting during EF-G-catalyzed translocation.Nat Commun. 2021 Jul 30;12(1):4644. doi: 10.1038/s41467-021-24911-1. Nat Commun. 2021. PMID: 34330903 Free PMC article.
-
Structural insights into mRNA reading frame regulation by tRNA modification and slippery codon-anticodon pairing.Elife. 2020 Oct 5;9:e51898. doi: 10.7554/eLife.51898. Elife. 2020. PMID: 33016876 Free PMC article.
-
Active role of elongation factor G in maintaining the mRNA reading frame during translation.Sci Adv. 2019 Dec 20;5(12):eaax8030. doi: 10.1126/sciadv.aax8030. eCollection 2019 Dec. Sci Adv. 2019. PMID: 31903418 Free PMC article.
-
Genome Expansion by tRNA +1 Frameshifting at Quadruplet Codons.J Mol Biol. 2022 Apr 30;434(8):167440. doi: 10.1016/j.jmb.2021.167440. Epub 2022 Jan 4. J Mol Biol. 2022. PMID: 34995554 Free PMC article. Review.
-
The many faces of ribosome translocation along the mRNA: reading frame maintenance, ribosome frameshifting and translational bypassing.Biol Chem. 2023 Apr 21;404(8-9):755-767. doi: 10.1515/hsz-2023-0142. Print 2023 Jul 26. Biol Chem. 2023. PMID: 37077160 Review.
Cited by
-
Modulation of translational decoding by m6A modification of mRNA.Nat Commun. 2023 Aug 8;14(1):4784. doi: 10.1038/s41467-023-40422-7. Nat Commun. 2023. PMID: 37553384 Free PMC article.
-
Streptomyces rare codon UUA: from features associated with 2 adpA related locations to candidate phage regulatory translational bypassing.RNA Biol. 2023 Jan;20(1):926-942. doi: 10.1080/15476286.2023.2270812. Epub 2023 Nov 15. RNA Biol. 2023. PMID: 37968863 Free PMC article.
-
Ribosomal frameshifting at normal codon repeats recodes functional chimeric proteins in human.Nucleic Acids Res. 2024 Mar 21;52(5):2463-2479. doi: 10.1093/nar/gkae035. Nucleic Acids Res. 2024. PMID: 38281188 Free PMC article.
References
-
- Moomau C, Musalgaonkar S, Khan YA, Jones JE, Dinman JD. Structural and functional characterization of programmed ribosomal frameshift signals in west nile virus strains reveals high structural plasticity among cis-acting RNA elements. J. Biol. Chem. 2016;291:15788–15795. doi: 10.1074/jbc.M116.735613. - DOI - PMC - PubMed
Publication types
MeSH terms
Substances
LinkOut - more resources
Full Text Sources
Molecular Biology Databases