Nitric Oxide Attenuates Human Cytomegalovirus Infection yet Disrupts Neural Cell Differentiation and Tissue Organization
- PMID: 35862705
- PMCID: PMC9327702
- DOI: 10.1128/jvi.00126-22
Nitric Oxide Attenuates Human Cytomegalovirus Infection yet Disrupts Neural Cell Differentiation and Tissue Organization
Abstract
Human cytomegalovirus (HCMV) is a prevalent betaherpesvirus that is asymptomatic in healthy individuals but can cause serious disease in immunocompromised patients. HCMV is also the leading cause of virus-mediated birth defects. Many of these defects manifest within the central nervous system and include microcephaly, sensorineural hearing loss, and cognitive developmental delays. Nitric oxide is a critical effector molecule produced as a component of the innate immune response during infection. Congenitally infected fetal brains show regions of brain damage, including necrotic foci with infiltrating macrophages and microglia, cell types that produce nitric oxide during infection. Using a 3-dimensional cortical organoid model, we demonstrate that nitric oxide inhibits HCMV spread and simultaneously disrupts neural rosette structures, resulting in tissue disorganization. Nitric oxide also attenuates HCMV replication in 2-dimensional cultures of neural progenitor cells (NPCs), a prominent cell type in cortical organoids that differentiate into neurons and glial cells. The multipotency factor SOX2 was decreased during nitric oxide exposure, suggesting that early neural differentiation is affected. Nitric oxide also reduced maximal mitochondrial respiration in both uninfected and infected NPCs. We determined that this reduction likely influences neural differentiation, as neurons (Tuj1+ GFAP- Nestin-) and glial populations (Tuj1- GFAP+ Nestin-) were reduced following differentiation. Our studies indicate a prominent, immunopathogenic role of nitric oxide in promoting developmental defects within the brain despite its antiviral activity during congenital HCMV infection. IMPORTANCE Human cytomegalovirus (HCMV) is the leading cause of virus-mediated congenital birth defects. Congenitally infected infants can have a variety of symptoms manifesting within the central nervous system. The use of 3-dimensional (3-D) cortical organoids to model infection of the fetal brain has advanced the current understanding of development and allowed broader investigation of the mechanisms behind disease. However, the impact of the innate immune molecule nitric oxide during HCMV infection has not been explored in neural cells or cortical 3-D models. Here, we investigated the effect of nitric oxide on cortical development during HCMV infection. We demonstrate that nitric oxide plays an antiviral role during infection yet results in disorganized cortical tissue. Nitric oxide contributes to differentiation defects of neuron and glial cells from neural progenitor cells despite inhibiting viral replication. Our results indicate that immunopathogenic consequences of nitric oxide during congenital infection promote developmental defects that undermine its antiviral activity.
Keywords: SOX2; cortical organoids; induced pluripotent stem cells; microcephaly; mitochondrial respiration; neural development; neural differentiation; neural progenitor cells.
Conflict of interest statement
The authors declare no conflict of interest.
Figures
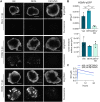
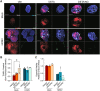
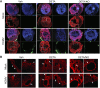
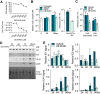
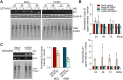
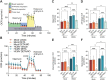
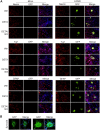
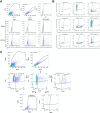
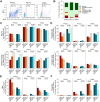
Similar articles
-
Human Cytomegalovirus Disruption of Calcium Signaling in Neural Progenitor Cells and Organoids.J Virol. 2019 Aug 13;93(17):e00954-19. doi: 10.1128/JVI.00954-19. Print 2019 Sep 1. J Virol. 2019. PMID: 31217241 Free PMC article.
-
Human Cytomegalovirus Compromises Development of Cerebral Organoids.J Virol. 2019 Aug 13;93(17):e00957-19. doi: 10.1128/JVI.00957-19. Print 2019 Sep 1. J Virol. 2019. PMID: 31217239 Free PMC article.
-
Nitric Oxide Circumvents Virus-Mediated Metabolic Regulation during Human Cytomegalovirus Infection.mBio. 2020 Dec 15;11(6):e02630-20. doi: 10.1128/mBio.02630-20. mBio. 2020. PMID: 33323506 Free PMC article.
-
Stem cell-based region-specific brain organoids: Novel models to understand neurodevelopmental defects.Birth Defects Res. 2022 Oct 1;114(16):1003-1013. doi: 10.1002/bdr2.2004. Epub 2022 Mar 25. Birth Defects Res. 2022. PMID: 35332709 Review.
-
Congenital cytomegalovirus infection undermines early development and functions of the human placenta.Placenta. 2017 Nov;59 Suppl 1:S8-S16. doi: 10.1016/j.placenta.2017.04.020. Epub 2017 Apr 25. Placenta. 2017. PMID: 28477968 Review.
Cited by
-
HCMV Infection Reduces Nidogen-1 Expression, Contributing to Impaired Neural Rosette Development in Brain Organoids.J Virol. 2023 May 31;97(5):e0171822. doi: 10.1128/jvi.01718-22. Epub 2023 May 1. J Virol. 2023. PMID: 37125912 Free PMC article.
-
Pathophysiology and mechanisms of hearing impairment related to neonatal infection diseases.Front Microbiol. 2023 Apr 14;14:1162554. doi: 10.3389/fmicb.2023.1162554. eCollection 2023. Front Microbiol. 2023. PMID: 37125179 Free PMC article. Review.
-
Stabilizing microtubules aids neurite structure and disrupts syncytia formation in human cytomegalovirus-infected human forebrain neurons.bioRxiv [Preprint]. 2024 Aug 19:2024.08.16.608340. doi: 10.1101/2024.08.16.608340. bioRxiv. 2024. PMID: 39229072 Free PMC article. Preprint.
-
Human pluripotent stem cell (hPSC) and organoid models of autism: opportunities and limitations.Transl Psychiatry. 2023 Jun 21;13(1):217. doi: 10.1038/s41398-023-02510-6. Transl Psychiatry. 2023. PMID: 37344450 Free PMC article. Review.
-
The Inhibition Effects of Sodium Nitroprusside on the Survival of Differentiated Neural Stem Cells through the p38 Pathway.Brain Sci. 2023 Mar 3;13(3):438. doi: 10.3390/brainsci13030438. Brain Sci. 2023. PMID: 36979248 Free PMC article.
References
-
- Shenk TE, Stinski MF. 2008. Human cytomegalovirus. Preface. Curr Top Microbiol Immunol 325:v. - PubMed
Publication types
MeSH terms
Substances
Grants and funding
LinkOut - more resources
Full Text Sources
Medical
Miscellaneous