Evidence for Dual Activation of IK(M) and IK(Ca) Caused by QO-58 (5-(2,6-Dichloro-5-fluoropyridin-3-yl)-3-phenyl-2-(trifluoromethyl)-1H-pyrazolol[1,5-a]pyrimidin-7-one)
- PMID: 35806047
- PMCID: PMC9266432
- DOI: 10.3390/ijms23137042
Evidence for Dual Activation of IK(M) and IK(Ca) Caused by QO-58 (5-(2,6-Dichloro-5-fluoropyridin-3-yl)-3-phenyl-2-(trifluoromethyl)-1H-pyrazolol[1,5-a]pyrimidin-7-one)
Abstract
QO-58 (5-(2,6-dichloro-5-fluoropyridin-3-yl)-3-phenyl-2-(trifluoromethyl)-1H-pyrazolol[1,5-a]pyrimidin-7-one) has been regarded to be an activator of KV7 channels with analgesic properties. However, whether and how the presence of this compound can result in any modifications of other types of membrane ion channels in native cells are not thoroughly investigated. In this study, we investigated its perturbations on M-type K+ current (IK(M)), Ca2+-activated K+ current (IK(Ca)), large-conductance Ca2+-activated K+ (BKCa) channels, and erg-mediated K+ current (IK(erg)) identified from pituitary tumor (GH3) cells. Addition of QO-58 can increase the amplitude of IK(M) and IK(Ca) in a concentration-dependent fashion, with effective EC50 of 3.1 and 4.2 μM, respectively. This compound could shift the activation curve of IK(M) toward a leftward direction with being void of changes in the gating charge. The strength in voltage-dependent hysteresis (Vhys) of IK(M) evoked by upright triangular ramp pulse (Vramp) was enhanced by adding QO-58. The probabilities of M-type K+ (KM) channels that will be open increased upon the exposure to QO-58, although no modification in single-channel conductance was seen. Furthermore, GH3-cell exposure to QO-58 effectively increased the amplitude of IK(Ca) as well as enhanced the activity of BKCa channels. Under inside-out configuration, QO-58, applied at the cytosolic leaflet of the channel, activated BKCa-channel activity, and its increase could be attenuated by further addition of verruculogen, but not by linopirdine (10 μM). The application of QO-58 could lead to a leftward shift in the activation curve of BKCa channels with neither change in the gating charge nor in single-channel conductance. Moreover, cell exposure of QO-58 (10 μM) resulted in a minor suppression of IK(erg) amplitude in response to membrane hyperpolarization. The docking results also revealed that there are possible interactions of the QO-58 molecule with the KCNQ or KCa1.1 channel. Overall, dual activation of IK(M) and IK(Ca) caused by the presence of QO-58 eventually may have high impacts on the functional activity (e.g., anti-nociceptive effect) residing in electrically excitable cells. Care must be exercised when interpreting data generated with QO-58 as it is not entirely KCNQ/KV7 selective.
Keywords: 5-(2,6-dichloro-5-fluoro-3-pyridinyl)-3-phenyl-2-(trifluoromethyl)-pyrazolo[1,5-a]pyrimidin-7(4H)-one); Ca2+-activated K+ current; M-type K+ channel; M-type K+ current; QO-58 (5-(2,6-dichloro-5-fluoropyridin-3-yl)-3-phenyl-2-(trifluoromethyl)-1H-pyrazolol[1,5-a]pyrimidin-7-one; large-conductance Ca2+-activated K+ channel; voltage-dependent hysteresis.
Conflict of interest statement
The authors declare no conflict of interests that are directly relevant to the present study. The content and writing of this paper are solely the responsibility of the authors.
Figures
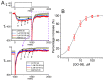
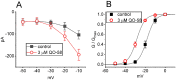
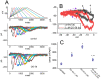
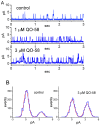
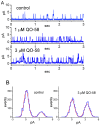
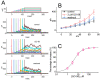
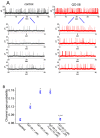
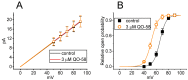
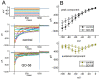
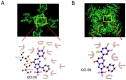
Similar articles
-
Effective Perturbations by Small-Molecule Modulators on Voltage-Dependent Hysteresis of Transmembrane Ionic Currents.Int J Mol Sci. 2022 Aug 21;23(16):9453. doi: 10.3390/ijms23169453. Int J Mol Sci. 2022. PMID: 36012718 Free PMC article. Review.
-
Effective Activation of BKCa Channels by QO-40 (5-(Chloromethyl)-3-(Naphthalen-1-yl)-2-(Trifluoromethyl)Pyrazolo [1,5-a]pyrimidin-7(4H)-one), Known to Be an Opener of KCNQ2/Q3 Channels.Pharmaceuticals (Basel). 2021 Apr 21;14(5):388. doi: 10.3390/ph14050388. Pharmaceuticals (Basel). 2021. PMID: 33919092 Free PMC article.
-
High Efficacy by GAL-021: A Known Intravenous Peripheral Chemoreceptor Modulator that Suppresses BKCa-Channel Activity and Inhibits IK(M) or Ih.Biomolecules. 2020 Jan 25;10(2):188. doi: 10.3390/biom10020188. Biomolecules. 2020. PMID: 31991782 Free PMC article.
-
The Effectiveness in Activating M-Type K+ Current Produced by Solifenacin ([(3R)-1-azabicyclo[2.2.2]octan-3-yl] (1S)-1-phenyl-3,4-dihydro-1H-isoquinoline-2-carboxylate): Independent of Its Antimuscarinic Action.Int J Mol Sci. 2021 Nov 17;22(22):12399. doi: 10.3390/ijms222212399. Int J Mol Sci. 2021. PMID: 34830281 Free PMC article.
-
Exploring the Impact of BKCa Channel Function in Cellular Membranes on Cardiac Electrical Activity.Int J Mol Sci. 2024 Jan 26;25(3):1537. doi: 10.3390/ijms25031537. Int J Mol Sci. 2024. PMID: 38338830 Free PMC article. Review.
Cited by
-
Ion Channels as a Potential Target in Pharmaceutical Designs.Int J Mol Sci. 2023 Mar 30;24(7):6484. doi: 10.3390/ijms24076484. Int J Mol Sci. 2023. PMID: 37047455 Free PMC article.
-
Effective Perturbations by Small-Molecule Modulators on Voltage-Dependent Hysteresis of Transmembrane Ionic Currents.Int J Mol Sci. 2022 Aug 21;23(16):9453. doi: 10.3390/ijms23169453. Int J Mol Sci. 2022. PMID: 36012718 Free PMC article. Review.
References
-
- Qi J., Zhang F., Mi Y., Fu Y., Xu W., Zhang D., Wu Y., Du X., Jia Q., Wang K., et al. Design, synthesis and biological activity of pyrazolo[1,5-a]pyrimidin-7(4H)-ones as novel Kv7/KCNQ potassium channel activators. Eur. J. Med. Chem. 2011;46:934–943. doi: 10.1016/j.ejmech.2011.01.010. - DOI - PubMed
-
- Teng B.-C., Song Y., Zhang F., Ma T.-Y., Qi J.-L., Zhang H.-L., Li G., Wang K. Activation of neuronal Kv7/KCNQ/M-channels by the opener QO58-lysine and its anti-nociceptive effects on inflammatory pain in rodents. Acta Pharmacol. Sin. 2016;37:1054–1062. doi: 10.1038/aps.2016.33. - DOI - PMC - PubMed
MeSH terms
Grants and funding
LinkOut - more resources
Full Text Sources
Medical
Miscellaneous