Phosphorylation of the small heat shock protein HspB1 regulates cytoskeletal recruitment and cell motility
- PMID: 35767320
- PMCID: PMC9582803
- DOI: 10.1091/mbc.E22-02-0057
Phosphorylation of the small heat shock protein HspB1 regulates cytoskeletal recruitment and cell motility
Abstract
The small heat shock protein HspB1, also known as Hsp25/27, is a ubiquitously expressed molecular chaperone that responds to mechanical cues. Uniaxial cyclic stretch activates the p38 mitogen-activated protein kinase (MAPK) signaling cascade and increases the phosphorylation of HspB1. Similar to the mechanosensitive cytoskeletal regulator zyxin, phospho-HspB1 is recruited to features of the stretch-stimulated actin cytoskeleton. To evaluate the role of HspB1 and its phosphoregulation in modulating cell function, we utilized CRISPR/Cas9-edited HspB1-null cells and determined they were altered in behaviors such as actin cytoskeletal remodeling, cell spreading, and cell motility. In our model system, expression of WT HspB1, but not nonphosphorylatable HspB1, rescued certain characteristics of the HspB1-null cells including the enhanced cell motility of HspB1-null cells and the deficient actin reinforcement of stretch-stimulated HspB1-null cells. The recruitment of HspB1 to high-tension structures in geometrically constrained cells, such as actin comet tails emanating from focal adhesions, also required a phosphorylatable HspB1. We show that mechanical signals activate posttranslational regulation of the molecular chaperone, HspB1, and are required for normal cell behaviors including actin cytoskeletal remodeling, cell spreading, and cell migration.
Figures
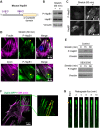
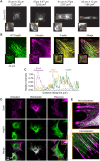
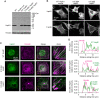
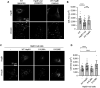
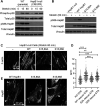
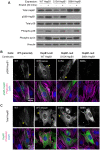
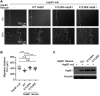
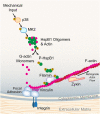
Similar articles
-
Mechanical signals activate p38 MAPK pathway-dependent reinforcement of actin via mechanosensitive HspB1.Mol Biol Cell. 2017 Oct 1;28(20):2661-2675. doi: 10.1091/mbc.E17-02-0087. Epub 2017 Aug 2. Mol Biol Cell. 2017. PMID: 28768826 Free PMC article.
-
HSPB1, actin filament dynamics, and aging cells.Ann N Y Acad Sci. 2010 Jun;1197:76-84. doi: 10.1111/j.1749-6632.2010.05191.x. Ann N Y Acad Sci. 2010. PMID: 20536836 Review.
-
Cell stress promotes the association of phosphorylated HspB1 with F-actin.PLoS One. 2013 Jul 10;8(7):e68978. doi: 10.1371/journal.pone.0068978. Print 2013. PLoS One. 2013. PMID: 23874834 Free PMC article.
-
The role of Hsp27 and actin in the regulation of movement in human cancer cells responding to heat shock.Cell Stress Chaperones. 2009 Sep;14(5):445-57. doi: 10.1007/s12192-008-0098-1. Epub 2009 Feb 18. Cell Stress Chaperones. 2009. PMID: 19224398 Free PMC article.
-
Mammalian HspB1 (Hsp27) is a molecular sensor linked to the physiology and environment of the cell.Cell Stress Chaperones. 2017 Jul;22(4):517-529. doi: 10.1007/s12192-017-0765-1. Epub 2017 Jan 31. Cell Stress Chaperones. 2017. PMID: 28144778 Free PMC article. Review.
Cited by
-
Mechanistic insights into heat shock protein 27, a potential therapeutic target for cardiovascular diseases.Front Cardiovasc Med. 2023 May 12;10:1195464. doi: 10.3389/fcvm.2023.1195464. eCollection 2023. Front Cardiovasc Med. 2023. PMID: 37252119 Free PMC article. Review.
-
From mesenchymal niches to engineered in vitro model systems: Exploring and exploiting biomechanical regulation of vertebrate hedgehog signalling.Mater Today Bio. 2022 Nov 22;17:100502. doi: 10.1016/j.mtbio.2022.100502. eCollection 2022 Dec 15. Mater Today Bio. 2022. PMID: 36457847 Free PMC article.
-
Functional Diversity of Mammalian Small Heat Shock Proteins: A Review.Cells. 2023 Jul 27;12(15):1947. doi: 10.3390/cells12151947. Cells. 2023. PMID: 37566026 Free PMC article. Review.
-
Time-resolved proximity proteomics uncovers a membrane tension-sensitive caveolin-1 interactome at the rear of migrating cells.Elife. 2024 Sep 24;13:e85601. doi: 10.7554/eLife.85601. Elife. 2024. PMID: 39315773 Free PMC article.
-
Regulation of Epithelial and Endothelial Barriers by Molecular Chaperones.Cells. 2024 Feb 21;13(5):370. doi: 10.3390/cells13050370. Cells. 2024. PMID: 38474334 Free PMC article. Review.
References
-
- Arber S, Hunter JJ, Ross J Jr, Hongo M, Sansig G, Borg J, Perriard JC, Chien KR, Caroni P (1997). MLP-deficient mice exhibit a disruption of cardiac cytoarchitectural organization, dilated cardiomyopathy, and heart failure. Cell 88, 393–403. - PubMed
Publication types
MeSH terms
Substances
Grants and funding
LinkOut - more resources
Full Text Sources
Research Materials
Miscellaneous