Impact of Cultured Neuron Models on α-Herpesvirus Latency Research
- PMID: 35746680
- PMCID: PMC9228292
- DOI: 10.3390/v14061209
Impact of Cultured Neuron Models on α-Herpesvirus Latency Research
Abstract
A signature trait of neurotropic α-herpesviruses (α-HV) is their ability to establish stable non-productive infections of peripheral neurons termed latency. This specialized gene expression program is the foundation of an evolutionarily successful strategy to ensure lifelong persistence in the host. Various physiological stresses can induce reactivation in a subset of latently-infected neurons allowing a new cycle of viral productive cycle gene expression and synthesis of infectious virus. Recurring reactivation events ensure transmission of the virus to new hosts and contributes to pathogenesis. Efforts to define the molecular basis of α-HV latency and reactivation have been notoriously difficult because the neurons harboring latent virus in humans and in experimentally infected live-animal models, are rare and largely inaccessible to study. Increasingly, researchers are turning to cultured neuron infection models as simpler experimental platforms from which to explore latency and reactivation at the molecular level. In this review, I reflect on the strengths and weaknesses of existing neuronal models and briefly summarize the important mechanistic insights these models have provided. I also discuss areas where prioritization will help to ensure continued progress and integration.
Keywords: HSV-1; HSV-2; VZV; cell culture; latency; neurons; reactivation.
Conflict of interest statement
The author declares no conflict of interest. The sponsors had no role in the conceptualization or writing of the manuscript.
Figures
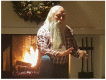
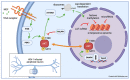
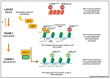
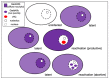
Similar articles
-
A primary neuron culture system for the study of herpes simplex virus latency and reactivation.J Vis Exp. 2012 Apr 2;(62):3823. doi: 10.3791/3823. J Vis Exp. 2012. PMID: 22491318 Free PMC article.
-
A comparison of herpes simplex virus type 1 and varicella-zoster virus latency and reactivation.J Gen Virol. 2015 Jul;96(Pt 7):1581-602. doi: 10.1099/vir.0.000128. Epub 2015 Mar 20. J Gen Virol. 2015. PMID: 25794504 Free PMC article. Review.
-
Lund Human Mesencephalic (LUHMES) Neuronal Cell Line Supports Herpes Simplex Virus 1 Latency In Vitro.J Virol. 2019 Mar 5;93(6):e02210-18. doi: 10.1128/JVI.02210-18. Print 2019 Mar 15. J Virol. 2019. PMID: 30602607 Free PMC article.
-
Modulation of Voltage-Gated Sodium Channel Activity in Human Dorsal Root Ganglion Neurons by Herpesvirus Quiescent Infection.J Virol. 2020 Jan 17;94(3):e01823-19. doi: 10.1128/JVI.01823-19. Print 2020 Jan 17. J Virol. 2020. PMID: 31694955 Free PMC article.
-
A cultured affair: HSV latency and reactivation in neurons.Trends Microbiol. 2012 Dec;20(12):604-11. doi: 10.1016/j.tim.2012.08.005. Epub 2012 Sep 7. Trends Microbiol. 2012. PMID: 22963857 Free PMC article. Review.
Cited by
-
Mouse Models for Human Herpesviruses.Pathogens. 2023 Jul 19;12(7):953. doi: 10.3390/pathogens12070953. Pathogens. 2023. PMID: 37513800 Free PMC article. Review.
-
Impaired glucocorticoid receptor function attenuates herpes simplex virus 1 production during explant-induced reactivation from latency in female mice.J Virol. 2023 Oct 31;97(10):e0130523. doi: 10.1128/jvi.01305-23. Epub 2023 Oct 12. J Virol. 2023. PMID: 37823644 Free PMC article.
-
Neuronal miR-9 promotes HSV-1 epigenetic silencing and latency by repressing Oct-1 and Onecut family genes.Nat Commun. 2024 Mar 5;15(1):1991. doi: 10.1038/s41467-024-46057-6. Nat Commun. 2024. PMID: 38443365 Free PMC article.
-
A cell cycle regulator, E2F2, and glucocorticoid receptor cooperatively transactivate the bovine alphaherpesvirus 1 immediate early transcription unit 1 promoter.J Virol. 2024 Jun 13;98(6):e0042324. doi: 10.1128/jvi.00423-24. Epub 2024 May 21. J Virol. 2024. PMID: 38771044 Free PMC article.
-
Intimate Relationship Between Stress and Human Alpha‑Herpes Virus 1 (HSV‑1) Reactivation from Latency.Curr Clin Microbiol Rep. 2023 Dec;10(4):236-245. doi: 10.1007/s40588-023-00202-9. Epub 2023 Jul 27. Curr Clin Microbiol Rep. 2023. PMID: 38173564 Free PMC article.
References
-
- Taylor M.W. A History of Cell Culture. In: Taylor M.W., editor. Viruses and Man: A History of Interactions. Springer International Publishing; Cham, Switzerland: 2014. pp. 41–52.
-
- Alberts B., Heald R., Johnson A., Morgan D., Raff M., Roberts K., Walter P. Molecular Biology of the Cell. 7th ed. W. W. Norton & Company; New York, NY, USA: 2022.
Publication types
MeSH terms
Grants and funding
LinkOut - more resources
Full Text Sources