FSHD Therapeutic Strategies: What Will It Take to Get to Clinic?
- PMID: 35743650
- PMCID: PMC9225474
- DOI: 10.3390/jpm12060865
FSHD Therapeutic Strategies: What Will It Take to Get to Clinic?
Abstract
Facioscapulohumeral muscular dystrophy (FSHD) is arguably one of the most challenging genetic diseases to understand and treat. The disease is caused by epigenetic dysregulation of a macrosatellite repeat, either by contraction of the repeat or by mutations in silencing proteins. Both cases lead to chromatin relaxation and, in the context of a permissive allele, pathogenic misexpression of DUX4 in skeletal muscle. The complex nature of the locus and the fact that FSHD is a toxic, gain-of-function disease present unique challenges for the design of therapeutic strategies. There are three major DUX4-targeting avenues of therapy for FSHD: small molecules, oligonucleotide therapeutics, and CRISPR-based approaches. Here, we evaluate the preclinical progress of each avenue, and discuss efforts being made to overcome major hurdles to translation.
Keywords: AAV; CRISPR; DUX4; FSHD; antisense; facioscapulohumeral muscular dystrophy; gene therapy; muscular dystrophy; skeletal muscle; therapeutics.
Conflict of interest statement
C.L.H. and P.L.J. are co-founders of EpiSwitch Rx, Inc., a company focused on bringing therapeutics for FSHD to the clinic. They are inventors on two U.S. patent applications pertaining to the use of CRISPR inhibition for FSHD and one pertaining to small molecule development for FSHD. P.L.J. is an inventor on a patent application identifying therapeutic targets for FSHD and on a patent for epigenetic diagnosis of FSHD.
Figures
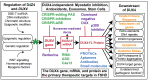
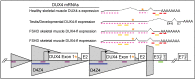
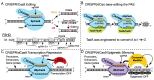
Similar articles
-
The Genetics and Epigenetics of Facioscapulohumeral Muscular Dystrophy.Annu Rev Genomics Hum Genet. 2019 Aug 31;20:265-291. doi: 10.1146/annurev-genom-083118-014933. Epub 2019 Apr 24. Annu Rev Genomics Hum Genet. 2019. PMID: 31018108 Review.
-
Targeted epigenetic repression by CRISPR/dSaCas9 suppresses pathogenic DUX4-fl expression in FSHD.Mol Ther Methods Clin Dev. 2020 Dec 10;20:298-311. doi: 10.1016/j.omtm.2020.12.001. eCollection 2021 Mar 12. Mol Ther Methods Clin Dev. 2020. PMID: 33511244 Free PMC article.
-
Identification of Epigenetic Regulators of DUX4-fl for Targeted Therapy of Facioscapulohumeral Muscular Dystrophy.Mol Ther. 2018 Jul 5;26(7):1797-1807. doi: 10.1016/j.ymthe.2018.04.019. Epub 2018 Apr 26. Mol Ther. 2018. PMID: 29759937 Free PMC article.
-
Antisense Oligonucleotides Used to Target the DUX4 mRNA as Therapeutic Approaches in FaciosScapuloHumeral Muscular Dystrophy (FSHD).Genes (Basel). 2017 Mar 3;8(3):93. doi: 10.3390/genes8030093. Genes (Basel). 2017. PMID: 28273791 Free PMC article.
-
Gene Editing to Tackle Facioscapulohumeral Muscular Dystrophy.Front Genome Ed. 2022 Jul 15;4:937879. doi: 10.3389/fgeed.2022.937879. eCollection 2022. Front Genome Ed. 2022. PMID: 35910413 Free PMC article. Review.
Cited by
-
The FSHD jigsaw: are we placing the tiles in the right position?Curr Opin Neurol. 2023 Oct 1;36(5):455-463. doi: 10.1097/WCO.0000000000001176. Epub 2023 Jun 14. Curr Opin Neurol. 2023. PMID: 37338810 Free PMC article. Review.
-
[Molecular therapies: present and future in neuromuscular diseases].Nervenarzt. 2023 Jun;94(6):473-487. doi: 10.1007/s00115-023-01495-3. Epub 2023 May 23. Nervenarzt. 2023. PMID: 37221259 Free PMC article. Review. German.
-
Influence of DUX4 Expression in Facioscapulohumeral Muscular Dystrophy and Possible Treatments.Int J Mol Sci. 2023 May 30;24(11):9503. doi: 10.3390/ijms24119503. Int J Mol Sci. 2023. PMID: 37298453 Free PMC article. Review.
-
The endosomal escape vehicle platform enhances delivery of oligonucleotides in preclinical models of neuromuscular disorders.Mol Ther Nucleic Acids. 2023 Jun 29;33:273-285. doi: 10.1016/j.omtn.2023.06.022. eCollection 2023 Sep 12. Mol Ther Nucleic Acids. 2023. PMID: 37538053 Free PMC article.
References
-
- Padberg G.W. Ph.D. Thesis. Leiden University; Leiden, The Netherlands: 1982. Facioscapulohumeral Disease.
-
- Orphanet Prevalence and Incidence of Rare Diseases: Bibliographic Data. Orphanet Report Series: Rare Diseases Collection. 2021. [(accessed on 23 May 2022)]. Available online: http://www.orpha.net/orphacom/cahiers/docs/GB/Prevalence_of_rare_disease....
Publication types
Grants and funding
LinkOut - more resources
Full Text Sources
Other Literature Sources