Single-Step Fast Tissue Clearing of Thick Mouse Brain Tissue for Multi-Dimensional High-Resolution Imaging
- PMID: 35743267
- PMCID: PMC9224586
- DOI: 10.3390/ijms23126826
Single-Step Fast Tissue Clearing of Thick Mouse Brain Tissue for Multi-Dimensional High-Resolution Imaging
Abstract
Recent advances in optical clearing techniques have dramatically improved deep tissue imaging by reducing the obscuring effects of light scattering and absorption. However, these optical clearing methods require specialized equipment or a lengthy undertaking with complex protocols that can lead to sample volume changes and distortion. In addition, the imaging of cleared tissues has limitations, such as fluorescence bleaching, harmful and foul-smelling solutions, and the difficulty of handling samples in high-viscosity refractive index (RI) matching solutions. To address the various limitations of thick tissue imaging, we developed an Aqueous high refractive Index matching and tissue Clearing solution for Imaging (termed AICI) with a one-step tissue clearing protocol that was easily made at a reasonable price in our own laboratory without any equipment. AICI can rapidly clear a 1 mm thick brain slice within 90 min with simultaneous RI matching, low viscosity, and a high refractive index (RI = 1.466), allowing the imaging of the sample without additional processing. We compared AICI with commercially available RI matching solutions, including optical clear agents (OCAs), for tissue clearing. The viscosity of AICI is closer to that of water compared with other RI matching solutions, and there was a less than 2.3% expansion in the tissue linear morphology during 24 h exposure to AICI. Moreover, AICI remained fluid over 30 days of air exposure, and the EGFP fluorescence signal was only reduced to ~65% after 10 days. AICI showed a limited clearing of brain tissue >3 mm thick. However, fine neuronal structures, such as dendritic spines and axonal boutons, could still be imaged in thick brain slices treated with AICI. Therefore, AICI is useful not only for the three-dimensional (3D) high-resolution identification of neuronal structures, but also for the examination of multiple structural imaging by neuronal distribution, projection, and gene expression in deep brain tissue. AICI is applicable beyond the imaging of fluorescent antibodies and dyes, and can clear a variety of tissue types, making it broadly useful to researchers for optical imaging applications.
Keywords: deep brain tissue; light sheet fluorescence microscopy; low viscosity; molecular imaging; refractive index matching solution; simple immersion; tissue clearing.
Conflict of interest statement
These authors declare no conflict of interest.
Figures
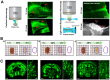
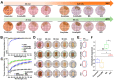
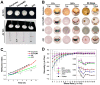
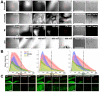
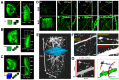
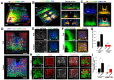
Similar articles
-
A method for ultrafast tissue clearing that preserves fluorescence for multimodal and longitudinal brain imaging.BMC Biol. 2022 Mar 29;20(1):77. doi: 10.1186/s12915-022-01275-6. BMC Biol. 2022. PMID: 35351101 Free PMC article.
-
Ultrafast optical clearing method for three-dimensional imaging with cellular resolution.Proc Natl Acad Sci U S A. 2019 Jun 4;116(23):11480-11489. doi: 10.1073/pnas.1819583116. Epub 2019 May 17. Proc Natl Acad Sci U S A. 2019. PMID: 31101714 Free PMC article.
-
Optical Clearing and Index Matching of Tissue Samples for High-resolution Fluorescence Imaging Using SeeDB2.Bio Protoc. 2018 Oct 20;8(20):e3046. doi: 10.21769/BioProtoc.3046. eCollection 2018 Oct 20. Bio Protoc. 2018. PMID: 34532520 Free PMC article.
-
Optical clearing methods: An overview of the techniques used for the imaging of 3D spheroids.Biotechnol Bioeng. 2019 Oct;116(10):2742-2763. doi: 10.1002/bit.27105. Epub 2019 Jul 21. Biotechnol Bioeng. 2019. PMID: 31282993 Review.
-
Optical clearing for multiscale biological tissues.J Biophotonics. 2018 Feb;11(2). doi: 10.1002/jbio.201700187. Epub 2017 Dec 12. J Biophotonics. 2018. PMID: 29024450 Review.
References
-
- Tsai P.S., Kaufhold J.P., Blinder P., Friedman B., Drew P.J., Karten H.J., Lyden P.D., Kleinfeld D. Correlations of neuronal and microvascular densities in murine cortex revealed by direct counting and colocalization of nuclei and vessels. J. Neurosci. 2009;29:14553–14570. doi: 10.1523/JNEUROSCI.3287-09.2009. - DOI - PMC - PubMed
MeSH terms
Grants and funding
- NRF-2020R1F1A1058459/National Research Foundation of Korea
- H I14C1135/Ministry of Health and Welfare
- 22-BR-03-02/the KBRI research program through Korea Brain Research Institute funded by the Ministry of Science, ICT & Future Planning
- S3102652/Technological innovation R&D program (industry and laboratory collabo R&D project) of MSS
LinkOut - more resources
Full Text Sources