Markers of fungal translocation are elevated during post-acute sequelae of SARS-CoV-2 and induce NF-κB signaling
- PMID: 35727635
- PMCID: PMC9462470
- DOI: 10.1172/jci.insight.160989
Markers of fungal translocation are elevated during post-acute sequelae of SARS-CoV-2 and induce NF-κB signaling
Erratum in
-
Markers of fungal translocation are elevated during post-acute sequelae of SARS-CoV-2 and induce NF-κB signaling.JCI Insight. 2022 Sep 22;7(18):e164813. doi: 10.1172/jci.insight.164813. JCI Insight. 2022. PMID: 36134654 Free PMC article. No abstract available.
Abstract
Long COVID, a type of post-acute sequelae of SARS-CoV-2 (PASC), has been associated with sustained elevated levels of immune activation and inflammation. However, the mechanisms that drive this inflammation remain unknown. Inflammation during acute coronavirus disease 2019 could be exacerbated by microbial translocation (from the gut and/or lung) to blood. Whether microbial translocation contributes to inflammation during PASC is unknown. We did not observe a significant elevation in plasma markers of bacterial translocation during PASC. However, we observed higher levels of fungal translocation - measured as β-glucan, a fungal cell wall polysaccharide - in the plasma of individuals experiencing PASC compared with those without PASC or SARS-CoV-2-negative controls. The higher β-glucan correlated with higher inflammation and elevated levels of host metabolites involved in activating N-methyl-d-aspartate receptors (such as metabolites within the tryptophan catabolism pathway) with established neurotoxic properties. Mechanistically, β-glucan can directly induce inflammation by binding to myeloid cells (via Dectin-1) and activating Syk/NF-κB signaling. Using a Dectin-1/NF-κB reporter model, we found that plasma from individuals experiencing PASC induced higher NF-κB signaling compared with plasma from negative controls. This higher NF-κB signaling was abrogated by piceatannol (Syk inhibitor). These data suggest a potential targetable mechanism linking fungal translocation and inflammation during PASC.
Keywords: COVID-19; Tight junctions; Virology.
Conflict of interest statement
Figures
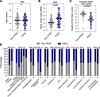
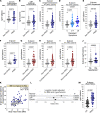
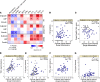
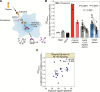
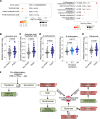
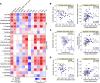
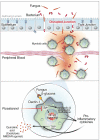
Similar articles
-
Activation of NF-κB and respiratory burst following Aspergillus fumigatus stimulation of macrophages.Immunobiology. 2014 Jan;219(1):25-36. doi: 10.1016/j.imbio.2013.06.013. Epub 2013 Jul 4. Immunobiology. 2014. PMID: 23886693
-
Tissue injury and leukocyte changes in post-acute sequelae of SARS-CoV-2: review of 2833 post-acute patient outcomes per immune dysregulation and microbial translocation in long COVID.J Leukoc Biol. 2023 Mar 1;113(3):236-254. doi: 10.1093/jleuko/qiac001. J Leukoc Biol. 2023. PMID: 36807444 Review.
-
Structure of a β-glucan from Grifola frondosa and its antitumor effect by activating Dectin-1/Syk/NF-κB signaling.Glycoconj J. 2012 Aug;29(5-6):365-77. doi: 10.1007/s10719-012-9416-z. Epub 2012 Jun 29. Glycoconj J. 2012. PMID: 22744837
-
The Neurological Manifestations of Post-Acute Sequelae of SARS-CoV-2 infection.Curr Neurol Neurosci Rep. 2021 Jun 28;21(9):44. doi: 10.1007/s11910-021-01130-1. Curr Neurol Neurosci Rep. 2021. PMID: 34181102 Free PMC article. Review.
-
Markers of Immune Activation and Inflammation in Individuals With Postacute Sequelae of Severe Acute Respiratory Syndrome Coronavirus 2 Infection.J Infect Dis. 2021 Dec 1;224(11):1839-1848. doi: 10.1093/infdis/jiab490. J Infect Dis. 2021. PMID: 34677601 Free PMC article.
Cited by
-
The microbiome in post-acute infection syndrome (PAIS).Comput Struct Biotechnol J. 2023 Aug 5;21:3904-3911. doi: 10.1016/j.csbj.2023.08.002. eCollection 2023. Comput Struct Biotechnol J. 2023. PMID: 37602232 Free PMC article. Review.
-
The gut-lung axis: the impact of the gut mycobiome on pulmonary diseases and infections.Oxf Open Immunol. 2024 Jul 24;5(1):iqae008. doi: 10.1093/oxfimm/iqae008. eCollection 2024. Oxf Open Immunol. 2024. PMID: 39193472 Free PMC article. Review.
-
Long COVID manifests with T cell dysregulation, inflammation, and an uncoordinated adaptive immune response to SARS-CoV-2.bioRxiv [Preprint]. 2023 Aug 4:2023.02.09.527892. doi: 10.1101/2023.02.09.527892. bioRxiv. 2023. Update in: Nat Immunol. 2024 Feb;25(2):218-225. doi: 10.1038/s41590-023-01724-6 PMID: 36798286 Free PMC article. Updated. Preprint.
-
Biomarkers in long COVID-19: A systematic review.Front Med (Lausanne). 2023 Jan 20;10:1085988. doi: 10.3389/fmed.2023.1085988. eCollection 2023. Front Med (Lausanne). 2023. PMID: 36744129 Free PMC article.
-
Researching COVID to Enhance Recovery (RECOVER) adult study protocol: Rationale, objectives, and design.PLoS One. 2023 Jun 23;18(6):e0286297. doi: 10.1371/journal.pone.0286297. eCollection 2023. PLoS One. 2023. PMID: 37352211 Free PMC article.