Tolerance of Senecavirus A to Mutations in Its Kissing-Loop or Pseudoknot Structure Computationally Predicted in 3' Untranslated Region
- PMID: 35707163
- PMCID: PMC9189406
- DOI: 10.3389/fmicb.2022.889480
Tolerance of Senecavirus A to Mutations in Its Kissing-Loop or Pseudoknot Structure Computationally Predicted in 3' Untranslated Region
Abstract
Senecavirus A (SVA) is an emerging virus that belongs to the genus Senecavirus in the family Picornaviridae. Its genome is a positive-sense and single-stranded RNA, containing two untranslated regions (UTRs). The 68-nt-long 3' UTR is computationally predicted to possess two higher-order RNA structures: a kissing-loop interaction and an H-type-like pseudoknot, both of which, however, cannot coexist in the 3' UTR. In this study, we constructed 17 full-length SVA cDNA clones (cD-1 to -17): the cD-1 to -7 contained different point mutations in a kissing-loop-forming motif (KLFM); the cD-8 to -17 harbored one single or multiple point mutations in a pseudoknot-forming motif (PFM). These 17 mutated cDNA clones were independently transfected into BSR-T7/5 cells for rescuing recombinant SVAs (rSVAs), named rSVA-1 to -17, corresponding to cD-1 to -17. The results showed that the rSVA-1, -2, -3, -4, -5, -6, -7, -9, -13, and -15 were successfully rescued from their individual cDNA clones. Moreover, all mutated motifs were genetically stable during 10 viral passages in vitro. This study unveiled viral abilities of tolerating mutations in the computationally predicted KLFM or PFMs. It can be concluded that the putative kissing-loop structure, even if present in the 3' UTR, is unnecessary for SVA replication. Alternatively, if the pseudoknot formation potentially occurs in the 3' UTR, its deformation would have a lethal effect on SVA propagation.
Keywords: 3′ untranslated region; Senecavirus A; kissing-loop; mutation; pseudoknot; reverse genetics.
Copyright © 2022 Liu, Zhao, Wang, Li, Dong, Liu, Zhang, Cui, Meng, Ni, Wei and Shan.
Conflict of interest statement
The authors declare that the research was conducted in the absence of any commercial or financial relationships that could be construed as a potential conflict of interest.
Figures
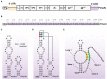
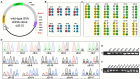
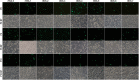
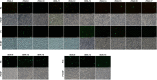
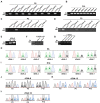
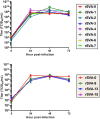
Similar articles
-
The 5'-end motif of Senecavirus A cDNA clone is genetically modified in 36 different ways for uncovering profiles of virus recovery.Front Microbiol. 2022 Aug 17;13:957849. doi: 10.3389/fmicb.2022.957849. eCollection 2022. Front Microbiol. 2022. PMID: 36060787 Free PMC article.
-
Motif mutations in pseudoknot stem I upstream of start codon in Senecavirus A genome: Impacts on activity of viral IRES and on rescue of recombinant virus.Vet Microbiol. 2021 Nov;262:109223. doi: 10.1016/j.vetmic.2021.109223. Epub 2021 Sep 2. Vet Microbiol. 2021. PMID: 34507016
-
Stem II-disrupting pseudoknot does not abolish ability of Senecavirus A IRES to initiate protein expression, but inhibits recovery of virus from cDNA clone.Vet Microbiol. 2021 Apr;255:109024. doi: 10.1016/j.vetmic.2021.109024. Epub 2021 Mar 2. Vet Microbiol. 2021. PMID: 33713975
-
[Structure and function of 3'- untranslated region in picornavirus].Bing Du Xue Bao. 2014 Jul;30(4):463-9. Bing Du Xue Bao. 2014. PMID: 25272604 Review. Chinese.
-
Senecavirus A.Vet Pathol. 2017 Jan;54(1):11-21. doi: 10.1177/0300985816653990. Epub 2016 Jul 11. Vet Pathol. 2017. PMID: 27371541 Review.
Cited by
-
The 5'-end motif of Senecavirus A cDNA clone is genetically modified in 36 different ways for uncovering profiles of virus recovery.Front Microbiol. 2022 Aug 17;13:957849. doi: 10.3389/fmicb.2022.957849. eCollection 2022. Front Microbiol. 2022. PMID: 36060787 Free PMC article.
-
The 3' end of the coding region of senecavirus A contains a highly conserved sequence that potentially forms a stem-loop structure required for virus rescue.Arch Virol. 2023 Sep 22;168(10):256. doi: 10.1007/s00705-023-05863-x. Arch Virol. 2023. PMID: 37737963
-
Only fourteen 3'-end poly(A)s sufficient for rescuing Senecavirus A from its cDNA clone, but inadequate to meet requirement of viral replication.Virus Res. 2023 Apr 15;328:199076. doi: 10.1016/j.virusres.2023.199076. Epub 2023 Mar 1. Virus Res. 2023. PMID: 36841440 Free PMC article.
References
-
- Buchholz U. J., Finke S., Conzelmann K. K. (1999). Generation of bovine respiratory syncytial virus (BRSV) from cDNA: BRSV NS2 is not essential for virus replication in tissue culture, and the human RSV leader region acts as a functional BRSV genome promoter. J. Virol. 73, 251–259. doi: 10.1128/JVI.73.1.251-259.1999, PMID: - DOI - PMC - PubMed
LinkOut - more resources
Full Text Sources
Research Materials