The Multidirectional Effect of Azelastine Hydrochloride on Cervical Cancer Cells
- PMID: 35682572
- PMCID: PMC9180047
- DOI: 10.3390/ijms23115890
The Multidirectional Effect of Azelastine Hydrochloride on Cervical Cancer Cells
Abstract
A major cause of cancer cell resistance to chemotherapeutics is the blocking of apoptosis and induction of autophagy in the context of cell adaptation and survival. Therefore, new compounds are being sought, also among drugs that are commonly used in other therapies. Due to the involvement of histamine in the regulation of processes occurring during the development of many types of cancer, antihistamines are now receiving special attention. Our study concerned the identification of new mechanisms of action of azelastine hydrochloride, used in antiallergic treatment. The study was performed on HeLa cells treated with different concentrations of azelastine (15-90 µM). Cell cycle, level of autophagy (LC3 protein activity) and apoptosis (annexin V assay), activity of caspase 3/7, anti-apoptotic protein of Bcl-2 family, ROS concentration, measurement of mitochondrial membrane potential (Δψm), and level of phosphorylated H2A.X in response to DSB were evaluated by cytometric method. Cellular changes were also demonstrated at the level of transmission electron microscopy and optical and fluorescence microscopy. Lysosomal enzyme activities-cathepsin D and L and cell viability (MTT assay) were assessed spectrophotometrically. Results: Azelastine in concentrations of 15-25 µM induced degradation processes, vacuolization, increase in cathepsin D and L activity, and LC3 protein activation. By increasing ROS, it also caused DNA damage and blocked cells in the S phase of the cell cycle. At the concentrations of 45-90 µM, azelastine clearly promoted apoptosis by activation of caspase 3/7 and inactivation of Bcl-2 protein. Fragmentation of cell nucleus was confirmed by DAPI staining. Changes were also found in the endoplasmic reticulum and mitochondria, whose damage was confirmed by staining with rhodamine 123 and in the MTT test. Azelastine decreased the mitotic index and induced mitotic catastrophe. Studies demonstrated the multidirectional effects of azelastine on HeLa cells, including anti-proliferative, cytotoxic, autophagic, and apoptotic properties, which were the predominant mechanism of death. The revealed novel properties of azelastine may be practically used in anti-cancer therapy in the future.
Keywords: apoptosis; autophagy; azelastine; mitotic catastrophe; oxidative stress.
Conflict of interest statement
The authors declare no conflict of interest.
Figures
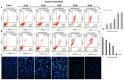
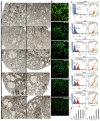
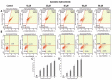
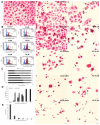
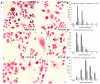
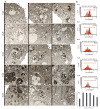
Similar articles
-
Rhein induces changes in the lysosomal compartment of HeLa cells.J Cell Biochem. 2022 Sep;123(9):1506-1524. doi: 10.1002/jcb.30311. Epub 2022 Jul 28. J Cell Biochem. 2022. PMID: 35901236
-
Physcion Induces Potential Anticancer Effects in Cervical Cancer Cells.Cells. 2021 Aug 8;10(8):2029. doi: 10.3390/cells10082029. Cells. 2021. PMID: 34440797 Free PMC article.
-
Emodin Sensitizes Cervical Cancer Cells to Vinblastine by Inducing Apoptosis and Mitotic Death.Int J Mol Sci. 2022 Jul 31;23(15):8510. doi: 10.3390/ijms23158510. Int J Mol Sci. 2022. PMID: 35955645 Free PMC article.
-
A novel trifluoromethyl benzopyran induces G1 cell cycle arrest and apoptosis in HeLa human cervical carcinoma cells.Int J Oncol. 2013 Aug;43(2):469-76. doi: 10.3892/ijo.2013.1958. Epub 2013 May 24. Int J Oncol. 2013. PMID: 23708884
-
ROS mediated ER stress induces Bax-Bak dependent and independent apoptosis in response to Thioridazine.Biomed Pharmacother. 2018 Oct;106:200-209. doi: 10.1016/j.biopha.2018.06.123. Epub 2018 Jun 28. Biomed Pharmacother. 2018. PMID: 29960166
Cited by
-
Chemical Stability Study of H1 Antihistaminic Drugs from the First and the Second Generations, Diphenhydramine, Azelastine and Bepotastine, in Pure APIs and in the Presence of Two Excipients, Citric Acid and Polyvinyl Alcohol.Molecules. 2022 Nov 29;27(23):8322. doi: 10.3390/molecules27238322. Molecules. 2022. PMID: 36500415 Free PMC article.
-
The mechanisms of action of mitochondrial targeting agents in cancer: inhibiting oxidative phosphorylation and inducing apoptosis.Front Pharmacol. 2023 Oct 25;14:1243613. doi: 10.3389/fphar.2023.1243613. eCollection 2023. Front Pharmacol. 2023. PMID: 37954849 Free PMC article. Review.
-
Lysosomes as a Target of Anticancer Therapy.Int J Mol Sci. 2023 Jan 22;24(3):2176. doi: 10.3390/ijms24032176. Int J Mol Sci. 2023. PMID: 36768500 Free PMC article. Review.
-
LHFPL2 Serves as a Potential Biomarker for M2 Polarization of Macrophages in Renal Cell Carcinoma.Int J Mol Sci. 2024 Jun 18;25(12):6707. doi: 10.3390/ijms25126707. Int J Mol Sci. 2024. PMID: 38928412 Free PMC article.
References
-
- Ellegaard A.M., Dehlendorff C., Vind A.C., Anand A., Cederkvist L., Petersen N.H.T., Nylandsted J., Stenvang J., Mellemgaard A., Osterlind K., et al. Repurposing Cationic Amphiphilic Antihistamines for Cancer Treatment. EBioMedicine. 2016;9:130–139. doi: 10.1016/j.ebiom.2016.06.013. - DOI - PMC - PubMed
MeSH terms
Substances
Grants and funding
LinkOut - more resources
Full Text Sources
Medical
Research Materials