Faulty autolysosome acidification in Alzheimer's disease mouse models induces autophagic build-up of Aβ in neurons, yielding senile plaques
- PMID: 35654956
- PMCID: PMC9174056
- DOI: 10.1038/s41593-022-01084-8
Faulty autolysosome acidification in Alzheimer's disease mouse models induces autophagic build-up of Aβ in neurons, yielding senile plaques
Abstract
Autophagy is markedly impaired in Alzheimer's disease (AD). Here we reveal unique autophagy dysregulation within neurons in five AD mouse models in vivo and identify its basis using a neuron-specific transgenic mRFP-eGFP-LC3 probe of autophagy and pH, multiplex confocal imaging and correlative light electron microscopy. Autolysosome acidification declines in neurons well before extracellular amyloid deposition, associated with markedly lowered vATPase activity and build-up of Aβ/APP-βCTF selectively within enlarged de-acidified autolysosomes. In more compromised yet still intact neurons, profuse Aβ-positive autophagic vacuoles (AVs) pack into large membrane blebs forming flower-like perikaryal rosettes. This unique pattern, termed PANTHOS (poisonous anthos (flower)), is also present in AD brains. Additional AVs coalesce into peri-nuclear networks of membrane tubules where fibrillar β-amyloid accumulates intraluminally. Lysosomal membrane permeabilization, cathepsin release and lysosomal cell death ensue, accompanied by microglial invasion. Quantitative analyses confirm that individual neurons exhibiting PANTHOS are the principal source of senile plaques in amyloid precursor protein AD models.
© 2022. The Author(s).
Conflict of interest statement
All authors declare no competing interests.
Figures
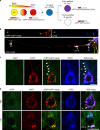
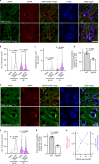
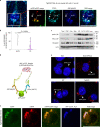
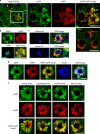
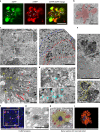
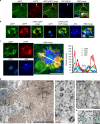
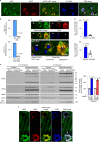
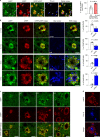
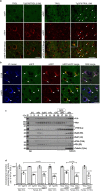
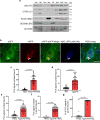
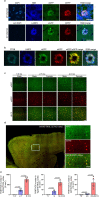
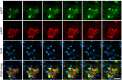
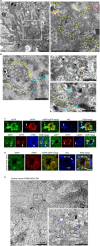
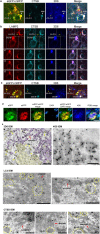
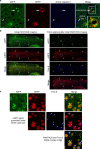
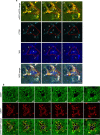
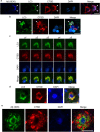
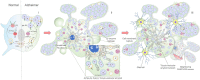
Comment in
-
Opening the box of PANTHORA in Alzheimer's disease.Signal Transduct Target Ther. 2022 Oct 2;7(1):344. doi: 10.1038/s41392-022-01204-7. Signal Transduct Target Ther. 2022. PMID: 36184585 Free PMC article. No abstract available.
Similar articles
-
Autolysosomal acidification failure as a primary driver of Alzheimer disease pathogenesis.Autophagy. 2022 Nov;18(11):2763-2764. doi: 10.1080/15548627.2022.2110729. Epub 2022 Aug 23. Autophagy. 2022. PMID: 35947489 Free PMC article.
-
Neuronal-Targeted TFEB Accelerates Lysosomal Degradation of APP, Reducing Aβ Generation and Amyloid Plaque Pathogenesis.J Neurosci. 2015 Sep 2;35(35):12137-51. doi: 10.1523/JNEUROSCI.0705-15.2015. J Neurosci. 2015. PMID: 26338325 Free PMC article.
-
Fibrillar Aβ triggers microglial proteome alterations and dysfunction in Alzheimer mouse models.Elife. 2020 Jun 8;9:e54083. doi: 10.7554/eLife.54083. Elife. 2020. PMID: 32510331 Free PMC article.
-
Autophagy-lysosomal-associated neuronal death in neurodegenerative disease.Acta Neuropathol. 2024 Sep 11;148(1):42. doi: 10.1007/s00401-024-02799-7. Acta Neuropathol. 2024. PMID: 39259382 Review.
-
Mechanisms of action of amyloid-beta and its precursor protein in neuronal cell death.Metab Brain Dis. 2020 Jan;35(1):11-30. doi: 10.1007/s11011-019-00516-y. Epub 2019 Dec 6. Metab Brain Dis. 2020. PMID: 31811496 Review.
Cited by
-
Mechanisms of autophagy-lysosome dysfunction in neurodegenerative diseases.Nat Rev Mol Cell Biol. 2024 Nov;25(11):926-946. doi: 10.1038/s41580-024-00757-5. Epub 2024 Aug 6. Nat Rev Mol Cell Biol. 2024. PMID: 39107446 Review.
-
Lysosome quality control in health and neurodegenerative diseases.Cell Mol Biol Lett. 2024 Sep 5;29(1):116. doi: 10.1186/s11658-024-00633-2. Cell Mol Biol Lett. 2024. PMID: 39237893 Free PMC article. Review.
-
An insight into the concept of neuroinflammation and neurodegeneration in Alzheimer's disease: targeting molecular approach Nrf2, NF-κB, and CREB.Inflammopharmacology. 2024 Oct;32(5):2943-2960. doi: 10.1007/s10787-024-01502-2. Epub 2024 Jun 29. Inflammopharmacology. 2024. PMID: 38951436 Review.
-
Rethinking of Alzheimer's disease: Lysosomal overloading and dietary therapy.Front Aging Neurosci. 2023 Feb 13;15:1130658. doi: 10.3389/fnagi.2023.1130658. eCollection 2023. Front Aging Neurosci. 2023. PMID: 36861123 Free PMC article. No abstract available.
-
The Pursuit of the "Inside" of the Amyloid Hypothesis-Is C99 a Promising Therapeutic Target for Alzheimer's Disease?Cells. 2023 Jan 31;12(3):454. doi: 10.3390/cells12030454. Cells. 2023. PMID: 36766796 Free PMC article. Review.
References
Publication types
MeSH terms
Substances
Grants and funding
LinkOut - more resources
Full Text Sources
Other Literature Sources
Medical
Molecular Biology Databases