Acute, Sublethal, and Developmental Toxicity of Kratom (Mitragyna speciosa Korth.) Leaf Preparations on Caenorhabditis elegans as an Invertebrate Model for Human Exposure
- PMID: 35627831
- PMCID: PMC9140534
- DOI: 10.3390/ijerph19106294
Acute, Sublethal, and Developmental Toxicity of Kratom (Mitragyna speciosa Korth.) Leaf Preparations on Caenorhabditis elegans as an Invertebrate Model for Human Exposure
Abstract
Kratom (Mitragyna speciosa Korth.) is a tree native to Southeast Asia with stimulant and opioid-like effects which has seen increased use in Europe and North America in recent years. Its safety and pharmacological effects remain under investigation, especially in regard to developmental and generational toxicity. In the current study, we investigated commercial kratom preparations using the nematode Caenorhabditis elegans as a translational model for toxicity and pharmacological effects. The pure alkaloids mitragynine and 7-hydroxymitragynine as well as aqueous, ethanolic, and methanolic extracts of three commercial kratom products were evaluated using a battery of developmental, genotoxic, and opioid-related experiments. As determined previously, the mitragynine and 7-hydroxymitragynine content in kratom samples was higher in the alcoholic extracts than the aqueous extracts. Above the human consumption range equivalent of 15-70 µg/mL, kratom dose-dependently reduced brood size and health of parent worms and their progeny. 7-hydroxymitragynine, but not mitragynine, presented with toxic and developmental effects at very high concentrations, while the positive control, morphine, displayed toxic effects at 0.5 mM. Kratom and its alkaloids did not affect pumping rate or interpump interval in the same way as morphine, suggesting that kratom is unlikely to act primarily via the opioid-signalling pathway. Only at very high doses did kratom cause developmental and genotoxic effects in nematodes, indicating its relative safety.
Keywords: body bending; opioid; pharyngeal pumping; reproduction; toxicity.
Conflict of interest statement
The authors declare no conflict of interest. The funders had no role in the design of the study; in the collection, analyses, or interpretation of data; in the writing of the manuscript; or in the decision to publish the results.
Figures
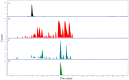
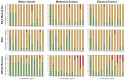
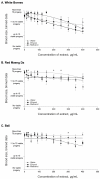
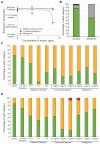
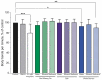
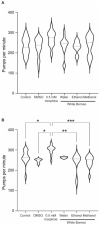
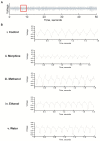
Similar articles
-
Correlations of kratom (Mitragyna speciosa Korth.) tea bag preparations and reported pharmacological effects.J Ethnopharmacol. 2023 Dec 5;317:116779. doi: 10.1016/j.jep.2023.116779. Epub 2023 Jun 24. J Ethnopharmacol. 2023. PMID: 37364801
-
Plant growth and phytoactive alkaloid synthesis in kratom [Mitragyna speciosa (Korth.)] in response to varying radiance.PLoS One. 2022 Apr 26;17(4):e0259326. doi: 10.1371/journal.pone.0259326. eCollection 2022. PLoS One. 2022. PMID: 35472200 Free PMC article.
-
Simultaneous quantification of ten key Kratom alkaloids in Mitragyna speciosa leaf extracts and commercial products by ultra-performance liquid chromatography-tandem mass spectrometry.Drug Test Anal. 2019 Aug;11(8):1162-1171. doi: 10.1002/dta.2604. Epub 2019 May 15. Drug Test Anal. 2019. PMID: 30997725 Free PMC article.
-
Beneficial and adverse health effects of kratom (Mitragyna speciosa): A critical review of the literature.Food Chem Toxicol. 2024 Oct;192:114913. doi: 10.1016/j.fct.2024.114913. Epub 2024 Aug 10. Food Chem Toxicol. 2024. PMID: 39134135 Review.
-
Kratom: Substance of Abuse or Therapeutic Plant?Psychiatr Clin North Am. 2022 Sep;45(3):415-430. doi: 10.1016/j.psc.2022.04.002. Epub 2022 Jul 31. Psychiatr Clin North Am. 2022. PMID: 36055730 Review.
Cited by
-
Exposure to silicone breast implant-infused media is detrimental to Caenorhabditis elegans.MicroPubl Biol. 2023 Feb 8;2023:10.17912/micropub.biology.000732. doi: 10.17912/micropub.biology.000732. eCollection 2023. MicroPubl Biol. 2023. PMID: 36855740 Free PMC article.
-
Kratom withdrawal: Discussions and conclusions of a scientific expert forum.Drug Alcohol Depend Rep. 2023 Mar 15;7:100142. doi: 10.1016/j.dadr.2023.100142. eCollection 2023 Jun. Drug Alcohol Depend Rep. 2023. PMID: 37397437 Free PMC article. No abstract available.
References
-
- Cinosi E., Martinotti G., Simanato P., Singh D., Demetrovics Z., Roman-Urrestarazu A., Saverio Bersani F., Vicknasingam B., Piazzon G., Li J.-H., et al. Following “the Roots” of Kratom (Mitragyna speciosa): The Evolution of an Enhancer from a Traditional Use to Increase Work and Productivity in Southeast Asia to a Recreational Psychoactive Drug in Western Countries. Biomed. Res. Int. 2015;2015:968786. doi: 10.1155/2015/968786. - DOI - PMC - PubMed
Publication types
MeSH terms
Substances
Grants and funding
LinkOut - more resources
Full Text Sources
Research Materials