The mechanism for thermal-enhanced chaperone-like activity of α-crystallin against UV irradiation-induced aggregation of γD-crystallin
- PMID: 35619565
- PMCID: PMC9279354
- DOI: 10.1016/j.bpj.2022.05.032
The mechanism for thermal-enhanced chaperone-like activity of α-crystallin against UV irradiation-induced aggregation of γD-crystallin
Abstract
Exposure to solar UV irradiation damages γ-crystallin, leading to cataract formation via aggregation. α-Crystallin, as a small heat shock protein, efficiently suppresses this irreversible aggregation by selectively binding the denatured γ-crystallin monomer. In this study, liquid chromatography tandem mass spectrometry was used to evaluate UV-325 nm irradiation-induced photodamage of human γD-crystallin in the presence of bovine α-crystallin, atomic force microscope (AFM) and dynamic light scattering (DLS) techniques were used to detect the quaternary structure changes of the α-crystallin oligomer, and Fourier transform infrared spectroscopy and temperature-jump nanosecond time-resolved IR absorbance difference spectroscopy were used to probe the secondary structure changes of bovine α-crystallin. We find that the thermal-induced subunit dissociation of the α-crystallin oligomer involves the breaking of hydrogen bonds at the dimeric interface, leading to three different spectral components at varied temperature regions as resolved from temperature-dependent IR spectra. Under UV-325 nm irradiation, unfolded γD-crystallin binds to the dissociated α-crystallin subunit to form an αγ-complex, then follows the reassociation of the αγ-complex to the partially dissociated α-crystallin oligomer. This prevents the aggregation of denatured γD-crystallin. The formation of the γD-bound α-crystallin oligomer is further confirmed by AFM and DLS analysis, which reveals an obvious size expansion in the reassociated αγ-oligomers. In addition, UV-325 nm irradiation causes a peptide bond cleavage of γD-crystallin at Ala158 in the presence of α-crystallin. Our results suggest a very effective protection mechanism for subunits dissociated from α-crystallin oligomers against UV irradiation-induced aggregation of γD-crystallin, at the expense of a loss of a short C-terminal peptide in γD-crystallin.
Copyright © 2022 Biophysical Society. Published by Elsevier Inc. All rights reserved.
Conflict of interest statement
Declaration of interests The authors declare no competing interests.
Figures
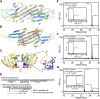
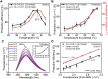
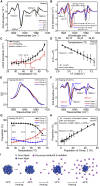
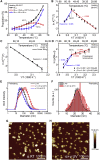
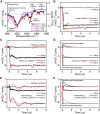
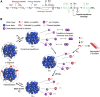
Similar articles
-
Partially folded aggregation intermediates of human gammaD-, gammaC-, and gammaS-crystallin are recognized and bound by human alphaB-crystallin chaperone.J Mol Biol. 2010 Aug 6;401(1):134-52. doi: 10.1016/j.jmb.2010.05.067. Epub 2010 Jun 1. J Mol Biol. 2010. PMID: 20621668 Free PMC article.
-
Mechanism of human γD-crystallin protein aggregation in UV-C light.Mol Vis. 2021 Jul 1;27:415-428. eCollection 2021. Mol Vis. 2021. PMID: 34267497 Free PMC article.
-
Cataract-causing defect of a mutant γ-crystallin proceeds through an aggregation pathway which bypasses recognition by the α-crystallin chaperone.PLoS One. 2012;7(5):e37256. doi: 10.1371/journal.pone.0037256. Epub 2012 May 24. PLoS One. 2012. PMID: 22655036 Free PMC article.
-
Chaperone-like activity of alpha-crystallin and other small heat shock proteins.Curr Protein Pept Sci. 2001 Sep;2(3):205-25. doi: 10.2174/1389203013381107. Curr Protein Pept Sci. 2001. PMID: 12369933 Review.
-
[The recent progress on the role of alpha-crystallin as a molecular chaperone in cataractogenesis].Yan Ke Xue Bao. 2000 Jun;16(2):91-6. Yan Ke Xue Bao. 2000. PMID: 12579912 Review. Chinese.
Cited by
-
Cold-induced calreticulin OsCRT3 conformational changes promote OsCIPK7 binding and temperature sensing in rice.EMBO J. 2023 Jan 4;42(1):e110518. doi: 10.15252/embj.2021110518. Epub 2022 Nov 7. EMBO J. 2023. PMID: 36341575 Free PMC article.
-
A neutral invertase controls cell division besides hydrolysis of sucrose for nutrition during germination and seed setting in rice.iScience. 2024 Jun 8;27(7):110217. doi: 10.1016/j.isci.2024.110217. eCollection 2024 Jul 19. iScience. 2024. PMID: 38993663 Free PMC article.
References
-
- De Jong W.W. In: Molecular and Cellular Biology of the Eye Lens. Bloemendal H., editor. John Wiley and Sons Inc.; New York: 1981. Evolution of lens and crystallins; pp. 221–278.
Publication types
MeSH terms
Substances
LinkOut - more resources
Full Text Sources
Miscellaneous