The Interplay Between Autophagy and RNA Homeostasis: Implications for Amyotrophic Lateral Sclerosis and Frontotemporal Dementia
- PMID: 35573690
- PMCID: PMC9096704
- DOI: 10.3389/fcell.2022.838402
The Interplay Between Autophagy and RNA Homeostasis: Implications for Amyotrophic Lateral Sclerosis and Frontotemporal Dementia
Abstract
Amyotrophic lateral sclerosis and frontotemporal dementia are neurodegenerative disorders that lie on a disease spectrum, sharing genetic causes and pathology, and both without effective therapeutics. Two pathways that have been shown to play major roles in disease pathogenesis are autophagy and RNA homeostasis. Intriguingly, there is an increasing body of evidence suggesting a critical interplay between these pathways. Autophagy is a multi-stage process for bulk and selective clearance of malfunctional cellular components, with many layers of regulation. Although the majority of autophagy research focuses on protein degradation, it can also mediate RNA catabolism. ALS/FTD-associated proteins are involved in many stages of autophagy and autophagy-mediated RNA degradation, particularly converging on the clearance of persistent pathological stress granules. In this review, we will summarise the progress in understanding the autophagy-RNA homeostasis interplay and how that knowledge contributes to our understanding of the pathobiology of ALS/FTD.
Keywords: C9orf72; RNA; RNA-binding proteins; amyotrophic lateral sclerosis; autophagy; frontotemporal dementia; granulophagy; stress granules.
Copyright © 2022 Houghton, Mizielinska and Gomez-Suaga.
Conflict of interest statement
The authors declare that the research was conducted in the absence of any commercial or financial relationships that could be construed as a potential conflict of interest.
Figures
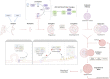
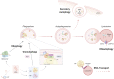
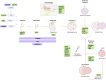
Similar articles
-
C9orf72 ALS-FTD: recent evidence for dysregulation of the autophagy-lysosome pathway at multiple levels.Autophagy. 2021 Nov;17(11):3306-3322. doi: 10.1080/15548627.2021.1872189. Epub 2021 Feb 26. Autophagy. 2021. PMID: 33632058 Free PMC article. Review.
-
Is amyotrophic lateral sclerosis/frontotemporal dementia an autophagy disease?Mol Neurodegener. 2017 Dec 28;12(1):90. doi: 10.1186/s13024-017-0232-6. Mol Neurodegener. 2017. PMID: 29282133 Free PMC article. Review.
-
Implications of Microglia in Amyotrophic Lateral Sclerosis and Frontotemporal Dementia.J Mol Biol. 2019 Apr 19;431(9):1818-1829. doi: 10.1016/j.jmb.2019.02.004. Epub 2019 Feb 11. J Mol Biol. 2019. PMID: 30763568 Review.
-
The converging roles of sequestosome-1/p62 in the molecular pathways of amyotrophic lateral sclerosis (ALS) and frontotemporal dementia (FTD).Neurobiol Dis. 2022 May;166:105653. doi: 10.1016/j.nbd.2022.105653. Epub 2022 Feb 7. Neurobiol Dis. 2022. PMID: 35143965 Review.
-
Pathomechanism Heterogeneity in the Amyotrophic Lateral Sclerosis and Frontotemporal Dementia Disease Spectrum: Providing Focus Through the Lens of Autophagy.J Mol Biol. 2020 Apr 3;432(8):2692-2713. doi: 10.1016/j.jmb.2020.02.018. Epub 2020 Feb 29. J Mol Biol. 2020. PMID: 32119873 Review.
Cited by
-
Frontotemporal Dementia, Where Do We Stand? A Narrative Review.Int J Mol Sci. 2023 Jul 21;24(14):11732. doi: 10.3390/ijms241411732. Int J Mol Sci. 2023. PMID: 37511491 Free PMC article. Review.
-
Janus kinase inhibitors are potential therapeutics for amyotrophic lateral sclerosis.Transl Neurodegener. 2023 Oct 12;12(1):47. doi: 10.1186/s40035-023-00380-y. Transl Neurodegener. 2023. PMID: 37828541 Free PMC article. Review.
References
-
- Al-Sarraj S., King A., Troakes C., Smith B., Maekawa S., Bodi I., et al. (2011). p62 Positive, TDP-43 Negative, Neuronal Cytoplasmic and Intranuclear Inclusions in the Cerebellum and Hippocampus Define the Pathology of C9orf72-Linked FTLD and MND/ALS. Acta Neuropathol. 122 (6), 691–702. 10.1007/s00401-011-0911-2 - DOI - PubMed
Publication types
LinkOut - more resources
Full Text Sources
Miscellaneous