Mucosal immune responses to infection and vaccination in the respiratory tract
- PMID: 35545027
- PMCID: PMC9087965
- DOI: 10.1016/j.immuni.2022.04.013
Mucosal immune responses to infection and vaccination in the respiratory tract
Abstract
The lungs are constantly exposed to inhaled debris, allergens, pollutants, commensal or pathogenic microorganisms, and respiratory viruses. As a result, innate and adaptive immune responses in the respiratory tract are tightly regulated and are in continual flux between states of enhanced pathogen clearance, immune-modulation, and tissue repair. New single-cell-sequencing techniques are expanding our knowledge of airway cellular complexity and the nuanced connections between structural and immune cell compartments. Understanding these varied interactions is critical in treatment of human pulmonary disease and infections and in next-generation vaccine design. Here, we review the innate and adaptive immune responses in the lung and airways following infection and vaccination, with particular focus on influenza virus and severe acute respiratory syndrome coronavirus 2 (SARS-CoV-2). The ongoing SARS-CoV-2 pandemic has put pulmonary research firmly into the global spotlight, challenging previously held notions of respiratory immunity and helping identify new populations at high risk for respiratory distress.
Copyright © 2022 Elsevier Inc. All rights reserved.
Conflict of interest statement
Declaration of interests P.G.T. has consulted or received honorarium and/or travel support from Illumina, JNJ, and 10X. P.G.T. serves on the Scientific Advisory Board of ImmunoScape and CytoAgents.
Figures
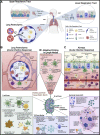
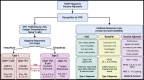
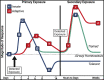
Similar articles
-
Mucosal Immunity against SARS-CoV-2 in the Respiratory Tract.Pathogens. 2024 Jan 26;13(2):113. doi: 10.3390/pathogens13020113. Pathogens. 2024. PMID: 38392851 Free PMC article. Review.
-
Human Nasal and Lung Tissues Infected Ex Vivo with SARS-CoV-2 Provide Insights into Differential Tissue-Specific and Virus-Specific Innate Immune Responses in the Upper and Lower Respiratory Tract.J Virol. 2021 Jun 24;95(14):e0013021. doi: 10.1128/JVI.00130-21. Epub 2021 Jun 24. J Virol. 2021. PMID: 33893170 Free PMC article.
-
Oral Bacteria Combined with an Intranasal Vaccine Protect from Influenza A Virus and SARS-CoV-2 Infection.mBio. 2021 Aug 31;12(4):e0159821. doi: 10.1128/mBio.01598-21. Epub 2021 Aug 17. mBio. 2021. PMID: 34399617 Free PMC article.
-
Insights into COVID-19 Vaccine Development Based on Immunogenic Structural Proteins of SARS-CoV-2, Host Immune Responses, and Herd Immunity.Cells. 2021 Oct 29;10(11):2949. doi: 10.3390/cells10112949. Cells. 2021. PMID: 34831172 Free PMC article. Review.
-
Unmasking the potential of secretory IgA and its pivotal role in protection from respiratory viruses.Antiviral Res. 2024 Mar;223:105823. doi: 10.1016/j.antiviral.2024.105823. Epub 2024 Feb 6. Antiviral Res. 2024. PMID: 38331200 Review.
Cited by
-
Location versus ID: what matters to lung-resident memory T cells?Front Immunol. 2024 Feb 5;15:1355910. doi: 10.3389/fimmu.2024.1355910. eCollection 2024. Front Immunol. 2024. PMID: 38375476 Free PMC article.
-
Role of IgA1 protease-producing bacteria in SARS-CoV-2 infection and transmission: a hypothesis.mBio. 2024 Oct 16;15(10):e0083324. doi: 10.1128/mbio.00833-24. Epub 2024 Aug 29. mBio. 2024. PMID: 39207101 Free PMC article.
-
Intramuscular mRNA BNT162b2 vaccine against SARS-CoV-2 induces neutralizing salivary IgA.Front Immunol. 2023 Jan 30;13:933347. doi: 10.3389/fimmu.2022.933347. eCollection 2022. Front Immunol. 2023. PMID: 36798518 Free PMC article.
-
Intranasal influenza-vectored COVID-19 vaccines confer broad protection against SARS-CoV-2 XBB variants in hamsters.PNAS Nexus. 2024 May 3;3(5):pgae183. doi: 10.1093/pnasnexus/pgae183. eCollection 2024 May. PNAS Nexus. 2024. PMID: 38800610 Free PMC article.
-
Surface Modification of Biodegradable Microparticles with the Novel Host-Derived Immunostimulant CPDI-02 Significantly Increases Short-Term and Long-Term Mucosal and Systemic Antibodies against Encapsulated Protein Antigen in Young Naïve Mice after Respiratory Immunization.Pharmaceutics. 2022 Sep 1;14(9):1843. doi: 10.3390/pharmaceutics14091843. Pharmaceutics. 2022. PMID: 36145590 Free PMC article.
References
-
- El Agha E., Moiseenko A., Kheirollahi V., De Langhe S., Crnkovic S., Kwapiszewska G., Szibor M., Kosanovic D., Schwind F., Schermuly R.T., et al. Two-way conversion between Lipogenic and Myogenic fibroblastic phenotypes Marks the progression and resolution of lung fibrosis. Cell Stem Cell. 2017;20:261–273.e3. doi: 10.1016/J.STEM.2016.10.004. - DOI - PMC - PubMed
-
- Aguirre A., Blazquez-Prieto J., Amado-Rodriguez L., Lopez-Alonso I., Batalla-Solis E., Gonzalez-Lopez A., Sanchez-Perez M., Mayoral-Garcia C., Gutierrez-Fernandez A., Albaiceta G.M. Matrix metalloproteinase-14 triggers an anti-inflammatory proteolytic cascade in endotoxemia. J. Mol. Med. 2017;95:487–497. doi: 10.1007/s00109-017-1510-z. - DOI - PubMed
Publication types
MeSH terms
Grants and funding
LinkOut - more resources
Full Text Sources
Medical
Research Materials
Miscellaneous