Enhanced bioactivity and osteoinductivity of carboxymethyl chitosan/nanohydroxyapatite/graphene oxide nanocomposites
- PMID: 35542061
- PMCID: PMC9080497
- DOI: 10.1039/c8ra00383a
Enhanced bioactivity and osteoinductivity of carboxymethyl chitosan/nanohydroxyapatite/graphene oxide nanocomposites
Abstract
Tissue engineering approaches combine a bioscaffold with stem cells to provide biological substitutes that can repair bone defects and eventually improve tissue functions. The prospective bioscaffold should have good osteoinductivity. Surface chemical and roughness modifications are regarded as valuable strategies for developing bioscaffolds because of their positive effects on enhancing osteogenic differentiation. However, the synergistic combination of the two strategies is currently poorly studied. In this work, a nanoengineered scaffold with surface chemistry (oxygen-containing groups) and roughness (R q = 74.1 nm) modifications was fabricated by doping nanohydroxyapatite (nHA), chemically crosslinked graphene oxide (GO) and carboxymethyl chitosan (CMC). The biocompatibility and osteoinductivity of the nanoengineered CMC/nHA/GO scaffold was evaluated in vitro and in vivo, and the osteogenic differentiation mechanism of the nanoengineered scaffold was preliminarily investigated. Our data demonstrated that the enhanced osteoinductivity of CMC/nHA/GO may profit from the surface chemistry and roughness, which benefit the β1 integrin interactions with the extracellular matrix and activate the FAK-ERK signaling pathway to upregulate the expression of osteogenic special proteins. This study indicates that the nanocomposite scaffold with surface chemistry and roughness modifications could serve as a novel and promising bone substitute for tissue engineering.
This journal is © The Royal Society of Chemistry.
Conflict of interest statement
The authors declare no competing financial interests.
Figures
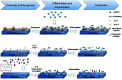
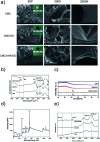
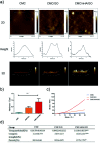
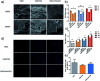
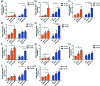
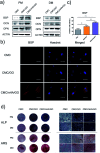
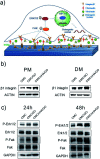
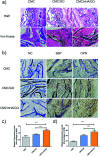
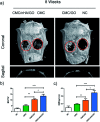
Similar articles
-
Reconstruction of Large-scale Defects with a Novel Hybrid Scaffold Made from Poly(L-lactic acid)/Nanohydroxyapatite/Alendronate-loaded Chitosan Microsphere: in vitro and in vivo Studies.Sci Rep. 2017 Mar 23;7(1):359. doi: 10.1038/s41598-017-00506-z. Sci Rep. 2017. PMID: 28337023 Free PMC article.
-
Biomimetic 3D-printed PCL scaffold containing a high concentration carbonated-nanohydroxyapatite with immobilized-collagen for bone tissue engineering: enhanced bioactivity and physicomechanical characteristics.Biomed Mater. 2021 Oct 29;16(6). doi: 10.1088/1748-605X/ac3147. Biomed Mater. 2021. PMID: 34670200
-
Novel chitosan/agarose/hydroxyapatite nanocomposite scaffold for bone tissue engineering applications: comprehensive evaluation of biocompatibility and osteoinductivity with the use of osteoblasts and mesenchymal stem cells.Int J Nanomedicine. 2019 Aug 19;14:6615-6630. doi: 10.2147/IJN.S217245. eCollection 2019. Int J Nanomedicine. 2019. PMID: 31695360 Free PMC article.
-
Recent trends in the application of widely used natural and synthetic polymer nanocomposites in bone tissue regeneration.Mater Sci Eng C Mater Biol Appl. 2020 May;110:110698. doi: 10.1016/j.msec.2020.110698. Epub 2020 Jan 29. Mater Sci Eng C Mater Biol Appl. 2020. PMID: 32204012 Free PMC article. Review.
-
Optimizing Nanohydroxyapatite Nanocomposites for Bone Tissue Engineering.ACS Omega. 2019 Dec 18;5(1):1-9. doi: 10.1021/acsomega.9b02917. eCollection 2020 Jan 14. ACS Omega. 2019. PMID: 31956745 Free PMC article. Review.
Cited by
-
Carbon Nanomaterial-Based Hydrogels as Scaffolds in Tissue Engineering: A Comprehensive Review.Int J Nanomedicine. 2023 Oct 27;18:6153-6183. doi: 10.2147/IJN.S436867. eCollection 2023. Int J Nanomedicine. 2023. PMID: 37915750 Free PMC article. Review.
-
A non-invasive smart scaffold for bone repair and monitoring.Bioact Mater. 2022 May 6;19:499-510. doi: 10.1016/j.bioactmat.2022.04.034. eCollection 2023 Jan. Bioact Mater. 2022. PMID: 35600976 Free PMC article.
-
Applications of Graphene and Its Derivatives in Bone Repair: Advantages for Promoting Bone Formation and Providing Real-Time Detection, Challenges and Future Prospects.Int J Nanomedicine. 2020 Oct 6;15:7523-7551. doi: 10.2147/IJN.S271917. eCollection 2020. Int J Nanomedicine. 2020. PMID: 33116486 Free PMC article. Review.
-
In Vitro and In Vivo Evaluation of Carboxymethyl Cellulose Scaffolds for Bone Tissue Engineering Applications.ACS Omega. 2021 Jan 4;6(2):1246-1253. doi: 10.1021/acsomega.0c04551. eCollection 2021 Jan 19. ACS Omega. 2021. PMID: 33490783 Free PMC article.
-
Structurally Tunable Reduced Graphene Oxide Substrate Maintains Mouse Embryonic Stem Cell Pluripotency.Adv Sci (Weinh). 2019 Apr 17;6(12):1802136. doi: 10.1002/advs.201802136. eCollection 2019 Jun 19. Adv Sci (Weinh). 2019. PMID: 31380157 Free PMC article.
References
-
- Pan T. Song W. Gao H. Li T. Cao X. Zhong S. Wang Y. ACS Appl. Mater. Interfaces. 2016;8:19217–19227. - PubMed
LinkOut - more resources
Full Text Sources
Miscellaneous