Use of standard U-bottom and V-bottom well plates to generate neuroepithelial embryoid bodies
- PMID: 35536781
- PMCID: PMC9089918
- DOI: 10.1371/journal.pone.0262062
Use of standard U-bottom and V-bottom well plates to generate neuroepithelial embryoid bodies
Abstract
The use of organoids has become increasingly popular recently due to their self-organizing abilities, which facilitate developmental and disease modeling. Various methods have been described to create embryoid bodies (EBs) generated from embryonic or pluripotent stem cells but with varying levels of differentiation success and producing organoids of variable size. Commercial ultra-low attachment (ULA) V-bottom well plates are frequently used to generate EBs. These plates are relatively expensive and not as widely available as standard concave well plates. Here, we describe a cost-effective and low labor-intensive method that creates homogeneous EBs at high yield in standard V- and U-bottom well plates by applying an anti-adherence solution to reduce surface attachment, followed by centrifugation to enhance cellular aggregation. We also explore the effect of different seeding densities, in the range of 1 to 11 ×103 cells per well, for the fabrication of neuroepithelial EBs. Our results show that the use of V-bottom well plates briefly treated with anti-adherent solution (for 5 min at room temperature) consistently yields functional neural EBs in the range of seeding densities from 5 to 11×103 cells per well. A brief post-seeding centrifugation step further enhances EB establishment. EBs fabricated using centrifugation exhibited lower variability in their final size than their non-centrifuged counterparts, and centrifugation also improved EB yield. The span of conditions for reliable EB production is narrower in U-bottom wells than in V-bottom wells (i.e., seeding densities between 7×103 and 11×103 and using a centrifugation step). We show that EBs generated by the protocols introduced here successfully developed into neural organoids and expressed the relevant markers associated with their lineages. We anticipate that the cost-effective and easily implemented protocols presented here will greatly facilitate the generation of EBs, thereby further democratizing the worldwide ability to conduct organoid-based research.
Conflict of interest statement
The authors have declared that no competing interests exist.
Figures
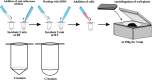
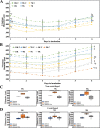
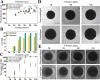
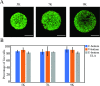
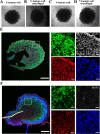
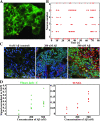
Similar articles
-
Formation of embryoid bodies from human pluripotent stem cells using AggreWell™ plates.Methods Mol Biol. 2013;946:523-33. doi: 10.1007/978-1-62703-128-8_32. Methods Mol Biol. 2013. PMID: 23179853
-
Cryobanking of embryoid bodies to facilitate basic research and cell-based therapies.Rejuvenation Res. 2011 Dec;14(6):641-9. doi: 10.1089/rej.2011.1186. Epub 2011 Oct 6. Rejuvenation Res. 2011. PMID: 21978080
-
Stress-free cell aggregation by using the CEPT cocktail enhances embryoid body and organoid fitness.Biofabrication. 2023 Dec 11;16(1). doi: 10.1088/1758-5090/ad0d13. Biofabrication. 2023. PMID: 37972398
-
Cell Mechanics in Embryoid Bodies.Cells. 2020 Oct 11;9(10):2270. doi: 10.3390/cells9102270. Cells. 2020. PMID: 33050550 Free PMC article. Review.
-
Properties of embryoid bodies.Wiley Interdiscip Rev Dev Biol. 2017 Mar;6(2). doi: 10.1002/wdev.259. Epub 2016 Dec 2. Wiley Interdiscip Rev Dev Biol. 2017. PMID: 27911036 Review.
Cited by
-
Brain organoids: A new tool for modelling of neurodevelopmental disorders.J Cell Mol Med. 2024 Sep;28(17):e18560. doi: 10.1111/jcmm.18560. J Cell Mol Med. 2024. PMID: 39258535 Free PMC article. Review.
-
Three-Dimensional Cell Cultures: The Bridge between In Vitro and In Vivo Models.Int J Mol Sci. 2023 Jul 27;24(15):12046. doi: 10.3390/ijms241512046. Int J Mol Sci. 2023. PMID: 37569426 Free PMC article. Review.
-
O2-sensitive microcavity arrays: A new platform for oxygen measurements in 3D cell cultures.Front Bioeng Biotechnol. 2023 Feb 20;11:1111316. doi: 10.3389/fbioe.2023.1111316. eCollection 2023. Front Bioeng Biotechnol. 2023. PMID: 36890915 Free PMC article.
-
The Analysis of Embryoid Body Formation and Its Role in Retinal Organoid Development.Int J Mol Sci. 2024 Jan 24;25(3):1444. doi: 10.3390/ijms25031444. Int J Mol Sci. 2024. PMID: 38338722 Free PMC article.
-
Morphological and molecular preservation through universal preparation of fresh-frozen tissue samples for multimodal imaging workflows.Nat Protoc. 2024 Sep;19(9):2685-2711. doi: 10.1038/s41596-024-00987-z. Epub 2024 May 28. Nat Protoc. 2024. PMID: 38806741 Review.
References
Publication types
MeSH terms
Grants and funding
LinkOut - more resources
Full Text Sources