Mechanisms of ribosome recycling in bacteria and mitochondria: a structural perspective
- PMID: 35485608
- PMCID: PMC9067457
- DOI: 10.1080/15476286.2022.2067712
Mechanisms of ribosome recycling in bacteria and mitochondria: a structural perspective
Abstract
In all living cells, the ribosome translates the genetic information carried by messenger RNAs (mRNAs) into proteins. The process of ribosome recycling, a key step during protein synthesis that ensures ribosomal subunits remain available for new rounds of translation, has been largely overlooked. Despite being essential to the survival of the cell, several mechanistic aspects of ribosome recycling remain unclear. In eubacteria and mitochondria, recycling of the ribosome into subunits requires the concerted action of the ribosome recycling factor (RRF) and elongation factor G (EF-G). Recently, the conserved protein HflX was identified in bacteria as an alternative factor that recycles the ribosome under stress growth conditions. The homologue of HflX, the GTP-binding protein 6 (GTPBP6), has a dual role in mitochondrial translation by facilitating ribosome recycling and biogenesis. In this review, mechanisms of ribosome recycling in eubacteria and mitochondria are described based on structural studies of ribosome complexes.
Keywords: GTPBP6; HflX; Ribosome; elongation factor G; protein synthesis; ribosome recycling; ribosome recycling factor; tRNA; translation factors.
Conflict of interest statement
No potential conflict of interest was reported by the author(s).
Figures
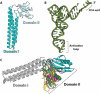
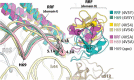
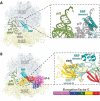
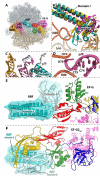
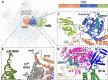
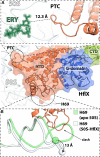
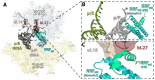
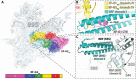
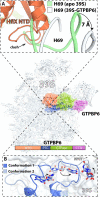
Similar articles
-
The hibernating 100S complex is a target of ribosome-recycling factor and elongation factor G in Staphylococcus aureus.J Biol Chem. 2020 May 1;295(18):6053-6063. doi: 10.1074/jbc.RA119.012307. Epub 2020 Mar 24. J Biol Chem. 2020. PMID: 32209660 Free PMC article.
-
Dual function of GTPBP6 in biogenesis and recycling of human mitochondrial ribosomes.Nucleic Acids Res. 2020 Dec 16;48(22):12929-12942. doi: 10.1093/nar/gkaa1132. Nucleic Acids Res. 2020. PMID: 33264405 Free PMC article.
-
New insights into the enzymatic role of EF-G in ribosome recycling.Nucleic Acids Res. 2015 Dec 2;43(21):10525-33. doi: 10.1093/nar/gkv995. Epub 2015 Oct 1. Nucleic Acids Res. 2015. PMID: 26432831 Free PMC article.
-
[Ribosome recycling revisited].Mol Biol (Mosk). 2006 Jul-Aug;40(4):742-50. Mol Biol (Mosk). 2006. PMID: 16913233 Review. Russian.
-
Dual functions of ribosome recycling factor in protein biosynthesis: disassembling the termination complex and preventing translational errors.Biochimie. 1996;78(11-12):959-69. doi: 10.1016/s0300-9084(97)86718-1. Biochimie. 1996. PMID: 9150873 Review.
Cited by
-
Special focus on the ribosome life cycle.RNA Biol. 2023 Jan;20(1):307-310. doi: 10.1080/15476286.2023.2221946. RNA Biol. 2023. PMID: 37312578 Free PMC article. No abstract available.
-
Mechanistic insights into the alternative ribosome recycling by HflXr.Nucleic Acids Res. 2024 Apr 24;52(7):4053-4066. doi: 10.1093/nar/gkae128. Nucleic Acids Res. 2024. PMID: 38407413 Free PMC article.
References
Publication types
MeSH terms
Substances
Grants and funding
LinkOut - more resources
Full Text Sources