Enzymatic Depletion of Mitochondrial Inorganic Polyphosphate (polyP) Increases the Generation of Reactive Oxygen Species (ROS) and the Activity of the Pentose Phosphate Pathway (PPP) in Mammalian Cells
- PMID: 35453370
- PMCID: PMC9029763
- DOI: 10.3390/antiox11040685
Enzymatic Depletion of Mitochondrial Inorganic Polyphosphate (polyP) Increases the Generation of Reactive Oxygen Species (ROS) and the Activity of the Pentose Phosphate Pathway (PPP) in Mammalian Cells
Abstract
Inorganic polyphosphate (polyP) is an ancient biopolymer that is well preserved throughout evolution and present in all studied organisms. In mammals, it shows a high co-localization with mitochondria, and it has been demonstrated to be involved in the homeostasis of key processes within the organelle, including mitochondrial bioenergetics. However, the exact extent of the effects of polyP on the regulation of cellular bioenergetics, as well as the mechanisms explaining these effects, still remain poorly understood. Here, using HEK293 mammalian cells under Wild-type (Wt) and MitoPPX (cells enzymatically depleted of mitochondrial polyP) conditions, we show that depletion of polyP within mitochondria increased oxidative stress conditions. This is characterized by enhanced mitochondrial O2- and intracellular H2O2 levels, which may be a consequence of the dysregulation of oxidative phosphorylation (OXPHOS) that we have demonstrated in MitoPPX cells in our previous work. These findings were associated with an increase in basal peroxiredoxin-1 (Prx1), superoxide dismutase-2 (SOD2), and thioredoxin (Trx) antioxidant protein levels. Using 13C-NMR and immunoblotting, we assayed the status of glycolysis and the pentose phosphate pathway (PPP) in Wt and MitoPPX cells. Our results show that MitoPPX cells display a significant increase in the activity of the PPP and an increase in the protein levels of transaldolase (TAL), which is a crucial component of the non-oxidative phase of the PPP and is involved in the regulation of oxidative stress. In addition, we observed a trend towards increased glycolysis in MitoPPX cells, which corroborates our prior work. Here, for the first time, we show the crucial role played by mitochondrial polyP in the regulation of mammalian redox homeostasis. Moreover, we demonstrate a significant effect of mitochondrial polyP on the regulation of global cellular bioenergetics in these cells.
Keywords: ROS; antioxidants; mammalian bioenergetics; mitochondria; mitochondrial inorganic polyphosphate; pentose phosphate pathway; polyP.
Conflict of interest statement
The authors declare no conflict of interest.
Figures
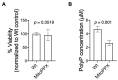
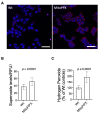
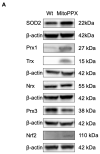
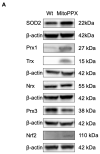
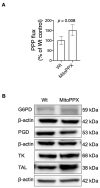
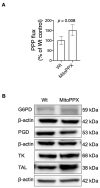
Similar articles
-
Toolkit for cellular studies of mammalian mitochondrial inorganic polyphosphate.Front Cell Dev Biol. 2023 Dec 15;11:1302585. doi: 10.3389/fcell.2023.1302585. eCollection 2023. Front Cell Dev Biol. 2023. PMID: 38161329 Free PMC article.
-
Mitochondrial Inorganic Polyphosphate (polyP) Is a Potent Regulator of Mammalian Bioenergetics in SH-SY5Y Cells: A Proteomics and Metabolomics Study.Front Cell Dev Biol. 2022 Feb 17;10:833127. doi: 10.3389/fcell.2022.833127. eCollection 2022. Front Cell Dev Biol. 2022. PMID: 35252194 Free PMC article.
-
Depletion of mitochondrial inorganic polyphosphate (polyP) in mammalian cells causes metabolic shift from oxidative phosphorylation to glycolysis.Biochem J. 2021 Apr 30;478(8):1631-1646. doi: 10.1042/BCJ20200975. Biochem J. 2021. PMID: 33843973 Free PMC article.
-
Is there a link between inorganic polyphosphate (polyP), mitochondria, and neurodegeneration?Pharmacol Res. 2021 Jan;163:105211. doi: 10.1016/j.phrs.2020.105211. Epub 2020 Oct 1. Pharmacol Res. 2021. PMID: 33010423 Free PMC article. Review.
-
Proliferating tumor cells mimick glucose metabolism of mature human erythrocytes.Cell Cycle. 2019 Jun;18(12):1316-1334. doi: 10.1080/15384101.2019.1618125. Epub 2019 Jun 3. Cell Cycle. 2019. PMID: 31154896 Free PMC article. Review.
Cited by
-
Mitochondrial inorganic polyphosphate is required to maintain proteostasis within the organelle.Front Cell Dev Biol. 2024 Jul 10;12:1423208. doi: 10.3389/fcell.2024.1423208. eCollection 2024. Front Cell Dev Biol. 2024. PMID: 39050895 Free PMC article.
-
Identification and prediction of association patterns between nutrient intake and anemia using machine learning techniques: results from a cross-sectional study with university female students from Palestine.Eur J Nutr. 2024 Aug;63(5):1635-1649. doi: 10.1007/s00394-024-03360-8. Epub 2024 Mar 21. Eur J Nutr. 2024. PMID: 38512358 Free PMC article.
-
Toolkit for cellular studies of mammalian mitochondrial inorganic polyphosphate.Front Cell Dev Biol. 2023 Dec 15;11:1302585. doi: 10.3389/fcell.2023.1302585. eCollection 2023. Front Cell Dev Biol. 2023. PMID: 38161329 Free PMC article.
-
The dynamic relationship between inorganic polyphosphate and adenosine triphosphate in human non-small cell lung cancer H1299 cells.FEBS Open Bio. 2024 Feb;14(2):344-354. doi: 10.1002/2211-5463.13753. Epub 2024 Jan 1. FEBS Open Bio. 2024. PMID: 38105501 Free PMC article.
-
Inorganic polyphosphate and the regulation of mitochondrial physiology.Biochem Soc Trans. 2023 Dec 20;51(6):2153-2161. doi: 10.1042/BST20230735. Biochem Soc Trans. 2023. PMID: 37955101 Free PMC article.
References
Grants and funding
LinkOut - more resources
Full Text Sources
Miscellaneous