Emergence of novel cephalopod gene regulation and expression through large-scale genome reorganization
- PMID: 35449136
- PMCID: PMC9023564
- DOI: 10.1038/s41467-022-29694-7
Emergence of novel cephalopod gene regulation and expression through large-scale genome reorganization
Abstract
Coleoid cephalopods (squid, cuttlefish, octopus) have the largest nervous system among invertebrates that together with many lineage-specific morphological traits enables complex behaviors. The genomic basis underlying these innovations remains unknown. Using comparative and functional genomics in the model squid Euprymna scolopes, we reveal the unique genomic, topological, and regulatory organization of cephalopod genomes. We show that coleoid cephalopod genomes have been extensively restructured compared to other animals, leading to the emergence of hundreds of tightly linked and evolutionary unique gene clusters (microsyntenies). Such novel microsyntenies correspond to topological compartments with a distinct regulatory structure and contribute to complex expression patterns. In particular, we identify a set of microsyntenies associated with cephalopod innovations (MACIs) broadly enriched in cephalopod nervous system expression. We posit that the emergence of MACIs was instrumental to cephalopod nervous system evolution and propose that microsyntenic profiling will be central to understanding cephalopod innovations.
© 2022. The Author(s).
Conflict of interest statement
The authors declare no competing interests.
Figures
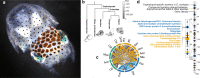
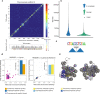
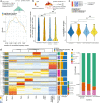
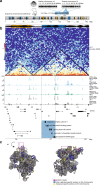
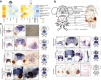
Similar articles
-
Genome and transcriptome mechanisms driving cephalopod evolution.Nat Commun. 2022 May 4;13(1):2427. doi: 10.1038/s41467-022-29748-w. Nat Commun. 2022. PMID: 35508532 Free PMC article.
-
Coupled Genomic Evolutionary Histories as Signatures of Organismal Innovations in Cephalopods: Co-evolutionary Signatures Across Levels of Genome Organization May Shed Light on Functional Linkage and Origin of Cephalopod Novelties.Bioessays. 2019 Dec;41(12):e1900073. doi: 10.1002/bies.201900073. Epub 2019 Oct 30. Bioessays. 2019. PMID: 31664724
-
The octopus genome and the evolution of cephalopod neural and morphological novelties.Nature. 2015 Aug 13;524(7564):220-4. doi: 10.1038/nature14668. Nature. 2015. PMID: 26268193 Free PMC article.
-
Evolution of the CT/CGRP family: comparative study with new data from models of teleosts, the eel, and cephalopod molluscs, the cuttlefish and the nautilus.Gen Comp Endocrinol. 2007 Aug-Sep;153(1-3):155-69. doi: 10.1016/j.ygcen.2007.01.027. Epub 2007 Jan 30. Gen Comp Endocrinol. 2007. PMID: 17353015 Review.
-
Cephalopod Biology: At the Intersection Between Genomic and Organismal Novelties.Annu Rev Anim Biosci. 2020 Feb 15;8:71-90. doi: 10.1146/annurev-animal-021419-083609. Epub 2019 Dec 9. Annu Rev Anim Biosci. 2020. PMID: 31815522 Review.
Cited by
-
Comparative genomics reveals insight into the evolutionary origin of massively scrambled genomes.Elife. 2022 Nov 24;11:e82979. doi: 10.7554/eLife.82979. Elife. 2022. PMID: 36421078 Free PMC article.
-
Deciphering regeneration through non-model animals: A century of experiments on cephalopod mollusks and an outlook at the future.Front Cell Dev Biol. 2023 Jan 9;10:1072382. doi: 10.3389/fcell.2022.1072382. eCollection 2022. Front Cell Dev Biol. 2023. PMID: 36699008 Free PMC article. Review.
-
Epigenetic machinery is functionally conserved in cephalopods.BMC Biol. 2022 Sep 14;20(1):202. doi: 10.1186/s12915-022-01404-1. BMC Biol. 2022. PMID: 36104784 Free PMC article.
-
Shifting landscapes: the role of 3D genomic organizations in gene regulatory strategies.Curr Opin Genet Dev. 2023 Aug;81:102064. doi: 10.1016/j.gde.2023.102064. Epub 2023 Jun 28. Curr Opin Genet Dev. 2023. PMID: 37390583 Free PMC article. Review.
-
Emergence of novel genomic regulatory regions associated with light-organ development in the bobtail squid.iScience. 2023 Jun 9;26(7):107091. doi: 10.1016/j.isci.2023.107091. eCollection 2023 Jul 21. iScience. 2023. PMID: 37426346 Free PMC article.
References
-
- Ritschard EA, et al. Coupled genomic evolutionary histories as signatures of organismal innovations in cephalopods: co-evolutionary signatures across levels of genome organization may shed light on functional linkage and origin of cephalopod novelties. BioEssays N. Rev. Mol. Cell. Dev. Biol. 2019;41:e1900073. - PubMed
Publication types
MeSH terms
Grants and funding
LinkOut - more resources
Full Text Sources