Mitochondrial protein translocation machinery: From TOM structural biogenesis to functional regulation
- PMID: 35346689
- PMCID: PMC9052162
- DOI: 10.1016/j.jbc.2022.101870
Mitochondrial protein translocation machinery: From TOM structural biogenesis to functional regulation
Abstract
The human mitochondrial outer membrane is biophysically unique as it is the only membrane possessing transmembrane β-barrel proteins (mitochondrial outer membrane proteins, mOMPs) in the cell. The most vital of the three mOMPs is the core protein of the translocase of the outer mitochondrial membrane (TOM) complex. Identified first as MOM38 in Neurospora in 1990, the structure of Tom40, the core 19-stranded β-barrel translocation channel, was solved in 2017, after nearly three decades. Remarkably, the past four years have witnessed an exponential increase in structural and functional studies of yeast and human TOM complexes. In addition to being conserved across all eukaryotes, the TOM complex is the sole ATP-independent import machinery for nearly all of the ∼1000 to 1500 known mitochondrial proteins. Recent cryo-EM structures have provided detailed insight into both possible assembly mechanisms of the TOM core complex and organizational dynamics of the import machinery and now reveal novel regulatory interplay with other mOMPs. Functional characterization of the TOM complex using biochemical and structural approaches has also revealed mechanisms for substrate recognition and at least five defined import pathways for precursor proteins. In this review, we discuss the discovery, recently solved structures, molecular function, and regulation of the TOM complex and its constituents, along with the implications these advances have for alleviating human diseases.
Keywords: TOM complex; Tom40; mitochondrial outer membrane; neurodegeneration; protein import pathways; transmembrane β-barrels.
Copyright © 2022 The Authors. Published by Elsevier Inc. All rights reserved.
Conflict of interest statement
Conflict of interest The authors declare that they no conflict of interest with the contents of this article.
Figures
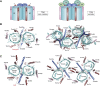
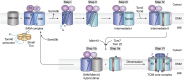
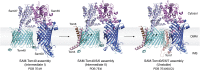
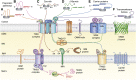
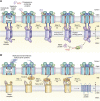
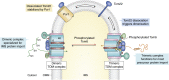
Similar articles
-
Role of the TOM Complex in Protein Import into Mitochondria: Structural Views.Annu Rev Biochem. 2022 Jun 21;91:679-703. doi: 10.1146/annurev-biochem-032620-104527. Epub 2022 Mar 14. Annu Rev Biochem. 2022. PMID: 35287471 Review.
-
Biogenesis of the mitochondrial TOM complex: Mim1 promotes insertion and assembly of signal-anchored receptors.J Biol Chem. 2008 Jan 4;283(1):120-127. doi: 10.1074/jbc.M706997200. Epub 2007 Nov 1. J Biol Chem. 2008. PMID: 17974559
-
Protein translocase of the outer mitochondrial membrane: role of import receptors in the structural organization of the TOM complex.J Mol Biol. 2002 Feb 22;316(3):657-66. doi: 10.1006/jmbi.2001.5365. J Mol Biol. 2002. PMID: 11866524
-
Two modular forms of the mitochondrial sorting and assembly machinery are involved in biogenesis of alpha-helical outer membrane proteins.J Mol Biol. 2010 Feb 26;396(3):540-9. doi: 10.1016/j.jmb.2009.12.026. Epub 2009 Dec 22. J Mol Biol. 2010. PMID: 20026336
-
The structure of the TOM core complex in the mitochondrial outer membrane.Biol Chem. 2020 May 26;401(6-7):687-697. doi: 10.1515/hsz-2020-0104. Biol Chem. 2020. PMID: 32142473 Review.
Cited by
-
Simple prerequisite of presequence for mitochondrial protein import in the unicellular red alga Cyanidioschyzon merolae.J Cell Sci. 2024 Jul 15;137(14):jcs262042. doi: 10.1242/jcs.262042. Epub 2024 Jul 23. J Cell Sci. 2024. PMID: 38940185 Free PMC article.
-
Gating of β-Barrel Protein Pores, Porins, and Channels: An Old Problem with New Facets.Int J Mol Sci. 2023 Jul 28;24(15):12095. doi: 10.3390/ijms241512095. Int J Mol Sci. 2023. PMID: 37569469 Free PMC article. Review.
-
The Role of PGC-1α-Mediated Mitochondrial Biogenesis in Neurons.Neurochem Res. 2023 Sep;48(9):2595-2606. doi: 10.1007/s11064-023-03934-8. Epub 2023 Apr 25. Neurochem Res. 2023. PMID: 37097395 Review.
-
Mitochondrial Dynamics and Mitophagy in Cardiometabolic Disease.Front Cardiovasc Med. 2022 Jun 17;9:917135. doi: 10.3389/fcvm.2022.917135. eCollection 2022. Front Cardiovasc Med. 2022. PMID: 35783853 Free PMC article. Review.
-
Photoradical-Mediated Catalyst-Independent Protein Cross-Link with Unusual Fluorescence Properties.Chembiochem. 2023 Sep 1;24(17):e202300380. doi: 10.1002/cbic.202300380. Epub 2023 Aug 1. Chembiochem. 2023. PMID: 37232210 Free PMC article.
References
-
- Roger A.J., Munoz-Gomez S.A., Kamikawa R. The origin and diversification of mitochondria. Curr. Biol. 2017;27:R1177–R1192. - PubMed
-
- Neupert W., Herrmann J.M. Translocation of proteins into mitochondria. Annu. Rev. Biochem. 2007;76:723–749. - PubMed
-
- Wiedemann N., Pfanner N. Mitochondrial machineries for protein import and assembly. Annu. Rev. Biochem. 2017;86:685–714. - PubMed
Publication types
MeSH terms
Substances
Grants and funding
LinkOut - more resources
Full Text Sources
Molecular Biology Databases
Miscellaneous