Microtubule polarity is instructive for many aspects of neuronal polarity
- PMID: 35341730
- PMCID: PMC9058238
- DOI: 10.1016/j.ydbio.2022.03.009
Microtubule polarity is instructive for many aspects of neuronal polarity
Abstract
Many neurons in bilaterian animals are polarized with functionally distinct axons and dendrites. Microtubule polarity, microtubule stability, and the axon initial segment (AIS) have all been shown to influence polarized transport in neurons. Each of these cytoskeletal cues could act independently to control axon and dendrite identity, or there could be a hierarchy in which one acts upstream of the others. Here we test the hypothesis that microtubule polarity acts as a master regulator of neuronal polarity by using a Drosophila genetic background in which some dendrites have normal minus-end-out microtubule polarity and others have the axonal plus-end-out polarity. In these mosaic dendrite arbors, we found that ribosomes, which are more abundant in dendrites than axons, were reduced in plus-end-out dendrites, while an axonal cargo was increased. In addition, we determined that microtubule stability was different in plus-end-out and minus-end-out dendrites, with plus-end-out ones having more stable microtubules like axons. Similarly, we found that ectopic diffusion barriers, like those at the AIS, formed at the base of dendrites with plus-end-out regions. Thus, changes in microtubule polarity were sufficient to rearrange other cytoskeletal features associated with neuronal polarization. However, overall neuron shape was maintained with only subtle changes in branching in mosaic arbors. We conclude that microtubule polarity can act upstream of many aspects of intracellular neuronal polarization, but shape is relatively resilient to changes in microtubule polarity in vivo.
Keywords: Axon; Axon initial segment; Dendrite; Microtubule polarity; Neuronal polarity.
Copyright © 2022 Elsevier Inc. All rights reserved.
Conflict of interest statement
Declaration of competing interest No competing interests are declared.
Figures
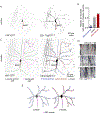
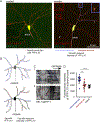
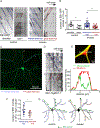
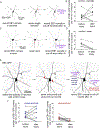
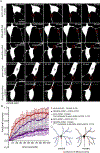
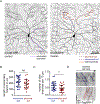
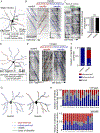
Similar articles
-
Dendrites In Vitro and In Vivo Contain Microtubules of Opposite Polarity and Axon Formation Correlates with Uniform Plus-End-Out Microtubule Orientation.J Neurosci. 2016 Jan 27;36(4):1071-85. doi: 10.1523/JNEUROSCI.2430-15.2016. J Neurosci. 2016. PMID: 26818498 Free PMC article.
-
Unrestrained growth of correctly oriented microtubules instructs axonal microtubule orientation.Elife. 2022 Oct 10;11:e77608. doi: 10.7554/eLife.77608. Elife. 2022. PMID: 36214669 Free PMC article.
-
Development of dendrite polarity in Drosophila neurons.Neural Dev. 2012 Oct 30;7:34. doi: 10.1186/1749-8104-7-34. Neural Dev. 2012. PMID: 23111238 Free PMC article.
-
Differentiation between Oppositely Oriented Microtubules Controls Polarized Neuronal Transport.Neuron. 2017 Dec 20;96(6):1264-1271.e5. doi: 10.1016/j.neuron.2017.11.018. Epub 2017 Nov 30. Neuron. 2017. PMID: 29198755 Free PMC article. Review.
-
Neuronal polarity: an evolutionary perspective.J Exp Biol. 2015 Feb 15;218(Pt 4):572-80. doi: 10.1242/jeb.112359. J Exp Biol. 2015. PMID: 25696820 Free PMC article. Review.
Cited by
-
Novel loss of function mutation in TUBA1A gene compromises tubulin stability and proteostasis causing spastic paraplegia and ataxia.Front Cell Neurosci. 2023 Jun 23;17:1162363. doi: 10.3389/fncel.2023.1162363. eCollection 2023. Front Cell Neurosci. 2023. PMID: 37435044 Free PMC article.
-
KLP-7/Kinesin-13 orchestrates axon-dendrite checkpoints for polarized trafficking in neurons.Mol Biol Cell. 2024 Sep 1;35(9):ar115. doi: 10.1091/mbc.E23-08-0335. Epub 2024 Jul 10. Mol Biol Cell. 2024. PMID: 38985513 Free PMC article.
-
Regulation of Cell Adhesion and Migration via Microtubule Cytoskeleton Organization, Cell Polarity, and Phosphoinositide Signaling.Biomolecules. 2023 Sep 22;13(10):1430. doi: 10.3390/biom13101430. Biomolecules. 2023. PMID: 37892112 Free PMC article. Review.
-
Minimal Mechanisms of Microtubule Length Regulation in Living Cells.Bull Math Biol. 2024 Apr 16;86(5):58. doi: 10.1007/s11538-024-01279-z. Bull Math Biol. 2024. PMID: 38627264
-
γ-TuRCs and the augmin complex are required for the development of highly branched dendritic arbors in Drosophila.J Cell Sci. 2024 May 1;137(9):jcs261534. doi: 10.1242/jcs.261534. Epub 2024 May 10. J Cell Sci. 2024. PMID: 38606636 Free PMC article.
References
-
- Baas PW, Slaughter T, Brown A and Black MM (1991). Microtubule dynamics in axons and dendrites. J Neurosci Res 30, 134–53. - PubMed
MeSH terms
Grants and funding
LinkOut - more resources
Full Text Sources
Molecular Biology Databases