Impact of Molecule Concentration, Diffusion Rates and Surface Passivation on Single-Molecule Fluorescence Studies in Solution
- PMID: 35327660
- PMCID: PMC8946791
- DOI: 10.3390/biom12030468
Impact of Molecule Concentration, Diffusion Rates and Surface Passivation on Single-Molecule Fluorescence Studies in Solution
Erratum in
-
Correction: Yukhnovets et al. Impact of Molecule Concentration, Diffusion Rates and Surface Passivation on Single-Molecule Fluorescence Studies in Solution. Biomolecules 2022, 12, 468.Biomolecules. 2024 Aug 14;14(8):1002. doi: 10.3390/biom14081002. Biomolecules. 2024. PMID: 39199430 Free PMC article.
Abstract
For single-molecule studies in solution, very small concentrations of dye-labelled molecules are employed in order to achieve single-molecule sensitivity. In typical studies with confocal microscopes, often concentrations in the pico-molar regime are required. For various applications that make use of single-molecule Förster resonance energy transfer (smFRET) or two-color coincidence detection (TCCD), the molecule concentration must be set explicitly to targeted values and furthermore needs to be stable over a period of several hours. As a consequence, specific demands must be imposed on the surface passivation of the cover slides during the measurements. The aim of having only one molecule in the detection volume at the time is not only affected by the absolute molecule concentration, but also by the rate of diffusion. Therefore, we discuss approaches to control and to measure absolute molecule concentrations. Furthermore, we introduce an approach to calculate the probability of chance coincidence events and demonstrate that measurements with challenging smFRET samples require a strict limit of maximal sample concentrations in order to produce meaningful results.
Keywords: burst analysis; chance coincidence probability; confocal fluorescence microscopy; single-molecule Förster resonance energy transfer; two-color coincidence detection.
Conflict of interest statement
The authors declare no conflict of interest.
Figures
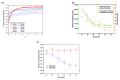
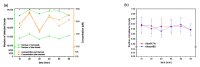
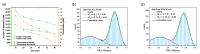
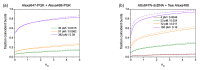
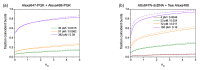
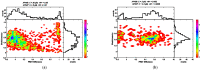
Similar articles
-
Probing the Conformational Landscape of DNA Polymerases Using Diffusion-Based Single-Molecule FRET.Methods Enzymol. 2016;581:353-378. doi: 10.1016/bs.mie.2016.08.023. Epub 2016 Oct 11. Methods Enzymol. 2016. PMID: 27793286
-
Structural Information from Single-molecule FRET Experiments Using the Fast Nano-positioning System.J Vis Exp. 2017 Feb 9;(120):54782. doi: 10.3791/54782. J Vis Exp. 2017. PMID: 28287526 Free PMC article.
-
Studying DNA-protein interactions with single-molecule Förster resonance energy transfer.Protoplasma. 2014 Mar;251(2):317-32. doi: 10.1007/s00709-013-0596-6. Epub 2013 Dec 28. Protoplasma. 2014. PMID: 24374460 Review.
-
FRETBursts: An Open Source Toolkit for Analysis of Freely-Diffusing Single-Molecule FRET.PLoS One. 2016 Aug 17;11(8):e0160716. doi: 10.1371/journal.pone.0160716. eCollection 2016. PLoS One. 2016. PMID: 27532626 Free PMC article.
-
The bright future of single-molecule fluorescence imaging.Curr Opin Chem Biol. 2014 Jun;20:103-11. doi: 10.1016/j.cbpa.2014.05.010. Epub 2014 Jun 21. Curr Opin Chem Biol. 2014. PMID: 24956235 Free PMC article. Review.
Cited by
-
The Thermodynamic Fingerprints of Ultra-Tight Nanobody-Antigen Binding Probed via Two-Color Single-Molecule Coincidence Detection.Int J Mol Sci. 2023 Nov 15;24(22):16379. doi: 10.3390/ijms242216379. Int J Mol Sci. 2023. PMID: 38003569 Free PMC article.
-
Correction: Yukhnovets et al. Impact of Molecule Concentration, Diffusion Rates and Surface Passivation on Single-Molecule Fluorescence Studies in Solution. Biomolecules 2022, 12, 468.Biomolecules. 2024 Aug 14;14(8):1002. doi: 10.3390/biom14081002. Biomolecules. 2024. PMID: 39199430 Free PMC article.
References
-
- Lerner E., Barth A., Hendrix J., Ambrose B., Birkedal V., Blanchard S.C., Borner R., Sung Chung H., Cordes T., Craggs T.D., et al. FRET-based dynamic structural biology: Challenges, perspectives and an appeal for open-science practices. Elife. 2021;10:e60416. doi: 10.7554/eLife.60416. - DOI - PMC - PubMed
-
- Sisamakis E., Valeri A., Kalinin S., Rothwell P.J., Seidel C.A. Accurate single-molecule FRET studies using multiparameter fluorescence detection. Methods Enzymol. 2010;475:455–514. - PubMed
-
- Deniz A.A., Laurence T.A., Beligere G.S., Dahan M., Martin A.B., Chemla D.S., Dawson P.E., Schultz P.G., Weiss S. Single-molecule protein folding: Diffusion fluorescence resonance energy transfer studies of the denaturation of chymotrypsin inhibitor 2. Proc. Natl. Acad. Sci. USA. 2000;97:5179–5184. doi: 10.1073/pnas.090104997. - DOI - PMC - PubMed
Publication types
MeSH terms
LinkOut - more resources
Full Text Sources
Research Materials