Telomerase RNA TERC and the PI3K-AKT pathway form a positive feedback loop to regulate cell proliferation independent of telomerase activity
- PMID: 35323972
- PMCID: PMC9023280
- DOI: 10.1093/nar/gkac179
Telomerase RNA TERC and the PI3K-AKT pathway form a positive feedback loop to regulate cell proliferation independent of telomerase activity
Abstract
The core catalytic unit of telomerase comprises telomerase reverse transcriptase (TERT) and telomerase RNA (TERC). Unlike TERT, which is predominantly expressed in cancer and stem cells, TERC is ubiquitously expressed in normal somatic cells without telomerase activity. However, the functions of TERC in these telomerase-negative cells remain elusive. Here, we reported positive feedback regulation between TERC and the PI3K-AKT pathway that controlled cell proliferation independent of telomerase activity in human fibroblasts. Mechanistically, we revealed that TERC activated the transcription of target genes from the PI3K-AKT pathway, such as PDPK1, by targeting their promoters. Overexpression of PDPK1 partially rescued the deficiency of AKT activation caused by TERC depletion. Furthermore, we found that FOXO1, a transcription factor negatively regulated by the PI3K-AKT pathway, bound to TERC promoter and suppressed its expression. Intriguingly, TERC-induced activation of the PI3K-AKT pathway also played a critical role in the proliferation of activated CD4+ T cells. Collectively, our findings identify a novel function of TERC that regulates the PI3K-AKT pathway via positive feedback to elevate cell proliferation independent of telomerase activity and provide a potential strategy to promote CD4+ T cells expansion that is responsible for enhancing adaptive immune reactions to defend against pathogens and tumor cells.
© The Author(s) 2022. Published by Oxford University Press on behalf of Nucleic Acids Research.
Figures
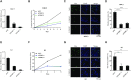
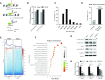
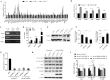
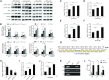
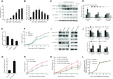
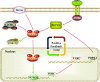
Similar articles
-
Telomerase enhances osteogenic ifferentiation of sheep bone marrow mesenchymal stem cells (BMSCs) by up-regulating PI3K/Akt pathway in vitro.Pol J Vet Sci. 2020 Sep;23(3):359-372. doi: 10.24425/pjvs.2020.133653. Pol J Vet Sci. 2020. PMID: 33006859
-
TERC promotes cellular inflammatory response independent of telomerase.Nucleic Acids Res. 2019 Sep 5;47(15):8084-8095. doi: 10.1093/nar/gkz584. Nucleic Acids Res. 2019. PMID: 31294790 Free PMC article.
-
POU2F2 regulates glycolytic reprogramming and glioblastoma progression via PDPK1-dependent activation of PI3K/AKT/mTOR pathway.Cell Death Dis. 2021 Apr 30;12(5):433. doi: 10.1038/s41419-021-03719-3. Cell Death Dis. 2021. PMID: 33931589 Free PMC article.
-
Noncanonical functions of telomerase and telomeres in viruses-associated cancer.J Med Virol. 2024 May;96(5):e29665. doi: 10.1002/jmv.29665. J Med Virol. 2024. PMID: 38738582 Review.
-
Increased copy number of the TERT and TERC telomerase subunit genes in cancer cells.Cancer Sci. 2008 Jun;99(6):1092-9. doi: 10.1111/j.1349-7006.2008.00815.x. Cancer Sci. 2008. PMID: 18482052 Free PMC article. Review.
Cited by
-
Lactic acid promotes nucleus pulposus cell senescence and corresponding intervertebral disc degeneration via interacting with Akt.Cell Mol Life Sci. 2024 Jan 12;81(1):24. doi: 10.1007/s00018-023-05094-y. Cell Mol Life Sci. 2024. PMID: 38212432 Free PMC article.
-
Many Functions of Telomerase Components: Certainties, Doubts, and Inconsistencies.Int J Mol Sci. 2022 Dec 2;23(23):15189. doi: 10.3390/ijms232315189. Int J Mol Sci. 2022. PMID: 36499514 Free PMC article. Review.
-
Prevalence and Associations of Co-occurrence of NFE2L2 Mutations and Chromosome 3q26 Amplification in Lung Cancer.Glob Med Genet. 2024 Apr 15;11(2):150-158. doi: 10.1055/s-0044-1786004. eCollection 2024 Jun. Glob Med Genet. 2024. PMID: 38628662 Free PMC article.
-
TIN2 modulates FOXO1 mitochondrial shuttling to enhance oxidative stress-induced apoptosis in retinal pigment epithelium under hyperglycemia.Cell Death Differ. 2024 Nov;31(11):1487-1505. doi: 10.1038/s41418-024-01349-8. Epub 2024 Jul 30. Cell Death Differ. 2024. PMID: 39080375
-
TERC suppresses PD-L1 expression by downregulating RNA binding protein HuR.Sci China Life Sci. 2022 Dec;65(12):2505-2516. doi: 10.1007/s11427-021-2085-9. Epub 2022 Jun 1. Sci China Life Sci. 2022. PMID: 35661964
References
MeSH terms
Substances
LinkOut - more resources
Full Text Sources
Molecular Biology Databases
Research Materials
Miscellaneous