Free fatty acid treatment of mouse preimplantation embryos demonstrates contrasting effects of palmitic acid and oleic acid on autophagy
- PMID: 35319901
- PMCID: PMC9273280
- DOI: 10.1152/ajpcell.00414.2021
Free fatty acid treatment of mouse preimplantation embryos demonstrates contrasting effects of palmitic acid and oleic acid on autophagy
Abstract
Treatment of mouse preimplantation embryos with elevated palmitic acid (PA) reduces blastocyst development, whereas cotreatment with PA and oleic acid (OA) together rescues blastocyst development to control frequencies. To understand the mechanistic effects of PA and OA treatment on early mouse embryos, we investigated the effects of PA and OA, alone and in combination, on autophagy during preimplantation development in vitro. We hypothesized that PA would alter autophagic processes and that OA cotreatment would restore control levels of autophagy. Two-cell stage mouse embryos were placed into culture medium supplemented with 100 μM PA, 250 μM OA, 100 μM PA and 250 μM OA, or potassium simplex optimization media with amino acid (KSOMaa) medium alone (control) for 18-48 h. The results demonstrated that OA cotreatment slowed developmental progression after 30 h of cotreatment but restored control blastocyst frequencies by 48 h. PA treatment elevated light chain 3 (LC3)-II puncta and p62 levels per cell whereas OA cotreatment returned to control levels of autophagy by 48 h. Autophagic mechanisms are altered by nonesterified fatty acid (NEFA) treatments during mouse preimplantation development in vitro, where PA elevates autophagosome formation and reduces autophagosome degradation levels, whereas cotreatment with OA reversed these PA effects. Autophagosome-lysosome colocalization only differed between PA and OA alone treatment groups. These findings advance our understanding of the effects of free fatty acid exposure on preimplantation development, and they uncover principles that may underlie the associations between elevated fatty acid levels and overall declines in reproductive fertility.
Keywords: autophagy; nonesterified fatty acids; obesity; preimplantation development; preimplantation embryos.
Conflict of interest statement
No conflicts of interest, financial or otherwise, are declared by the authors.
Figures
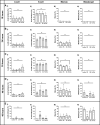
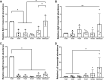
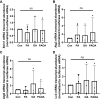
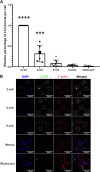
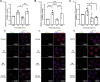
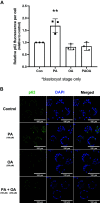
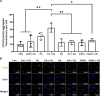
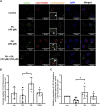
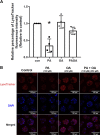
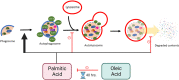
Similar articles
-
Oleic Acid Counters Impaired Blastocyst Development Induced by Palmitic Acid During Mouse Preimplantation Development: Understanding Obesity-Related Declines in Fertility.Reprod Sci. 2020 Nov;27(11):2038-2051. doi: 10.1007/s43032-020-00223-5. Epub 2020 Jun 15. Reprod Sci. 2020. PMID: 32542540 Free PMC article.
-
Expression and localization of NRF2/Keap1 signalling pathway genes in mouse preimplantation embryos exposed to free fatty acids.Gene Expr Patterns. 2022 Dec;46:119281. doi: 10.1016/j.gep.2022.119281. Epub 2022 Oct 13. Gene Expr Patterns. 2022. PMID: 36243294
-
Effects of palmitic acid on localization of embryo cell fate and blastocyst formation gene products.Reproduction. 2022 Feb 14;163(3):133-143. doi: 10.1530/REP-21-0354. Reproduction. 2022. PMID: 35038315 Free PMC article.
-
Oleic acid in the modulation of oocyte and preimplantation embryo development.Zygote. 2018 Feb;26(1):1-13. doi: 10.1017/S0967199417000582. Epub 2017 Dec 15. Zygote. 2018. PMID: 29244016 Review.
-
Fatty Acids: An Insight into the Pathogenesis of Neurodegenerative Diseases and Therapeutic Potential.Int J Mol Sci. 2022 Feb 25;23(5):2577. doi: 10.3390/ijms23052577. Int J Mol Sci. 2022. PMID: 35269720 Free PMC article. Review.
Cited by
-
Fatty acid supplementation during warming improves pregnancy outcomes after frozen blastocyst transfers: a propensity score-matched study.Sci Rep. 2024 Apr 23;14(1):9343. doi: 10.1038/s41598-024-60136-0. Sci Rep. 2024. PMID: 38653766 Free PMC article.
-
Low-input lipidomics reveals lipid metabolism remodelling during early mammalian embryo development.Nat Cell Biol. 2024 Feb;26(2):278-293. doi: 10.1038/s41556-023-01341-3. Epub 2024 Feb 1. Nat Cell Biol. 2024. PMID: 38302721
-
Autophagy-related gene and protein expressions during blastocyst development.J Assist Reprod Genet. 2023 Feb;40(2):323-331. doi: 10.1007/s10815-022-02698-4. Epub 2022 Dec 28. J Assist Reprod Genet. 2023. PMID: 36576685 Free PMC article.
References
-
- Ubags ND, Stapleton RD, Vernooy JH, Burg E, Bement J, Hayes CM, Ventrone S, Zabeau L, Tavernier J, Poynter ME, Parsons PE, Dixon AE, Wargo MJ, Littenberg B, Wouters EF, Suratt BT. Hyperleptinemia is associated with impaired pulmonary host defense. JCI Insight 1: e82101, 2016. doi:10.1172/jci.insight.82101. - DOI - PMC - PubMed
Publication types
MeSH terms
Substances
Grants and funding
LinkOut - more resources
Full Text Sources