Nanoparticle Delivery Platforms for RNAi Therapeutics Targeting COVID-19 Disease in the Respiratory Tract
- PMID: 35269550
- PMCID: PMC8909959
- DOI: 10.3390/ijms23052408
Nanoparticle Delivery Platforms for RNAi Therapeutics Targeting COVID-19 Disease in the Respiratory Tract
Abstract
Since December 2019, a pandemic of COVID-19 disease, caused by the severe acute respiratory syndrome coronavirus 2 (SARS-CoV-2), has rapidly spread across the globe. At present, the Food and Drug Administration (FDA) has issued emergency approval for the use of some antiviral drugs. However, these drugs still have limitations in the specific treatment of COVID-19, and as such, new treatment strategies urgently need to be developed. RNA-interference-based gene therapy provides a tractable target for antiviral treatment. Ensuring cell-specific targeted delivery is important to the success of gene therapy. The use of nanoparticles (NPs) as carriers for the delivery of small interfering RNA (siRNAs) to specific tissues or organs of the human body could play a crucial role in the specific therapy of severe respiratory infections, such as COVID-19. In this review, we describe a variety of novel nanocarriers, such as lipid NPs, star polymer NPs, and glycogen NPs, and summarize the pre-clinical/clinical progress of these nanoparticle platforms in siRNA delivery. We also discuss the application of various NP-capsulated siRNA as therapeutics for SARS-CoV-2 infection, the challenges with targeting these therapeutics to local delivery in the lung, and various inhalation devices used for therapeutic administration. We also discuss currently available animal models that are used for preclinical assessment of RNA-interference-based gene therapy. Advances in this field have the potential for antiviral treatments of COVID-19 disease and could be adapted to treat a range of respiratory diseases.
Keywords: COVID-19; glycogen nanoparticles; inhalation; lipid nanoparticles; nanomedicine; nanoparticle-capsulated drug delivery; polymer nanoparticles; siRNA.
Conflict of interest statement
The authors declare no conflict of interest.
Figures
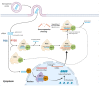
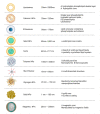
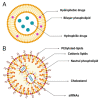
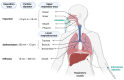
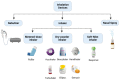
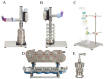
Similar articles
-
A SARS-CoV-2 targeted siRNA-nanoparticle therapy for COVID-19.Mol Ther. 2021 Jul 7;29(7):2219-2226. doi: 10.1016/j.ymthe.2021.05.004. Epub 2021 May 14. Mol Ther. 2021. PMID: 33992805 Free PMC article.
-
Bio-inspired materials in drug delivery: Exploring the role of pulmonary surfactant in siRNA inhalation therapy.J Control Release. 2015 Dec 28;220(Pt B):642-50. doi: 10.1016/j.jconrel.2015.09.004. Epub 2015 Sep 10. J Control Release. 2015. PMID: 26363301 Review.
-
Recent advances in siRNA delivery.Biomol Concepts. 2015 Dec;6(5-6):321-41. doi: 10.1515/bmc-2015-0019. Biomol Concepts. 2015. PMID: 26609865 Review.
-
Optimizing use of theranostic nanoparticles as a life-saving strategy for treating COVID-19 patients.Theranostics. 2020 May 1;10(13):5932-5942. doi: 10.7150/thno.46691. eCollection 2020. Theranostics. 2020. PMID: 32483428 Free PMC article. Review.
-
Lipid and Polymer-Based Nanoparticle siRNA Delivery Systems for Cancer Therapy.Molecules. 2020 Jun 10;25(11):2692. doi: 10.3390/molecules25112692. Molecules. 2020. PMID: 32532030 Free PMC article. Review.
Cited by
-
Liposomes and liposome-like nanoparticles: From anti-fungal infection to the COVID-19 pandemic treatment.Asian J Pharm Sci. 2022 Nov;17(6):817-837. doi: 10.1016/j.ajps.2022.11.002. Epub 2022 Nov 17. Asian J Pharm Sci. 2022. PMID: 36415834 Free PMC article. Review.
-
Improved Olfactory Deposition of Theophylline Using a Nanotech Soft Mist Nozzle Chip.Pharmaceutics. 2023 Dec 19;16(1):2. doi: 10.3390/pharmaceutics16010002. Pharmaceutics. 2023. PMID: 38276480 Free PMC article.
-
Nanomaterials to combat SARS-CoV-2: Strategies to prevent, diagnose and treat COVID-19.Front Bioeng Biotechnol. 2022 Nov 25;10:1052436. doi: 10.3389/fbioe.2022.1052436. eCollection 2022. Front Bioeng Biotechnol. 2022. PMID: 36507266 Free PMC article. Review.
-
Targeted Delivery to Dying Cells Through P-Selectin-PSGL-1 Axis: A Promising Strategy for Enhanced Drug Efficacy in Liver Injury Models.Cells. 2024 Oct 27;13(21):1778. doi: 10.3390/cells13211778. Cells. 2024. PMID: 39513885 Free PMC article.
-
Anticipating the Next Chess Move: Blocking SARS-CoV-2 Replication and Simultaneously Disarming Viral Escape Mechanisms.Genes (Basel). 2022 Nov 18;13(11):2147. doi: 10.3390/genes13112147. Genes (Basel). 2022. PMID: 36421821 Free PMC article.
References
-
- Counoupas C., Johansen M.D., Stella A.O., Nguyen D.H., Ferguson A.L., Aggarwal A., Bhattacharyya N.D., Grey A., Hutchings O., Patel K., et al. A single dose, BCG-adjuvanted COVID-19 vaccine provides sterilising immunity against SARS-CoV-2 infection. NPJ Vaccines. 2021;6:143. doi: 10.1038/s41541-021-00406-4. - DOI - PMC - PubMed
-
- Seow J., Graham C., Merrick B., Acors S., Pickering S., Steel K.J.A., Hemmings O., O’Byrne A., Kouphou N., Galao R.P., et al. Longitudinal observation and decline of neutralizing antibody responses in the three months following SARS-CoV-2 infection in humans. Nat. Microbiol. 2020;5:1598–1607. doi: 10.1038/s41564-020-00813-8. - DOI - PMC - PubMed
-
- Naaber P., Tserel L., Kangro K., Sepp E., Jurjenson V., Adamson A., Haljasmagi L., Rumm A.P., Maruste R., Karner J., et al. Dynamics of antibody response to BNT162b2 vaccine after six months: A longitudinal prospective study. Lancet Reg. Health Eur. 2021;10:100208. doi: 10.1016/j.lanepe.2021.100208. - DOI - PMC - PubMed
Publication types
MeSH terms
Substances
Grants and funding
LinkOut - more resources
Full Text Sources
Other Literature Sources
Medical
Miscellaneous