Three-dimensional printing of polycaprolactone/hydroxyapatite bone tissue engineering scaffolds mechanical properties and biological behavior
- PMID: 35267105
- PMCID: PMC8913482
- DOI: 10.1007/s10856-022-06653-8
Three-dimensional printing of polycaprolactone/hydroxyapatite bone tissue engineering scaffolds mechanical properties and biological behavior
Abstract
Controlled pore size and desirable internal architecture of bone scaffolds play a significant role in bone regeneration efficiency. In addition to choosing appropriate materials, the manufacturing method is another significant factor in fabricating the ideal scaffold. In this study, scaffolds were designed and fabricated by the fused filament fabrication (FFF) technique. Polycaprolactone (PCL) and composites films with various percentages of hydroxyapatite (HA) (up to 20%wt) were used to fabricate filaments. The influence of (HA) addition on the mechanical properties of filaments and scaffolds was investigated. in vitro biological evaluation was examined as well as the apatite formation in simulated body fluid (SBF). The addition of HA particles increased the compressive strength and Young's modulus of filaments and consequently the scaffolds. Compared to PCL, Young's modulus of PCL/HA20% filament and three-dimensional (3D) printed scaffold has increased by 30% and 50%, respectively. Also, Young's modulus for all scaffolds was in the range of 30-70 MPa, which is appropriate to use in spongy bone. Besides, the MTT assay was utilized to evaluate cell viability on the scaffolds. All the samples had qualified cytocompatibility, and it would be anticipated that addition of HA particles raise the biocompatibility in vivo. Alkaline phosphatase (ALP) evaluation shows that the addition of HA caused higher ALP activity in the PCL/HA scaffolds than PCL. Furthermore, calcium deposition in the PCL/HA specimens is higher than control. In conclusion, the addition of HA particles into the PCL matrix, as well as utilizing an inexpensive commercial FFF device, lead to the fabrication of scaffolds with proper mechanical and biological properties for bone tissue engineering applications. Graphical abstract.
© 2022. The Author(s).
Conflict of interest statement
The authors declare no competing interests.
Figures
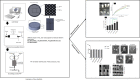
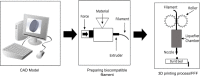
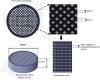
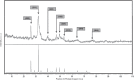
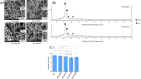
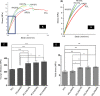
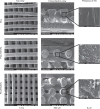
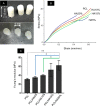
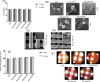

Similar articles
-
Towards Polycaprolactone-Based Scaffolds for Alveolar Bone Tissue Engineering: A Biomimetic Approach in a 3D Printing Technique.Int J Mol Sci. 2023 Nov 10;24(22):16180. doi: 10.3390/ijms242216180. Int J Mol Sci. 2023. PMID: 38003368 Free PMC article. Review.
-
Preparation and characterization of PLA/PCL/HA composite scaffolds using indirect 3D printing for bone tissue engineering.Mater Sci Eng C Mater Biol Appl. 2019 Nov;104:109960. doi: 10.1016/j.msec.2019.109960. Epub 2019 Jul 6. Mater Sci Eng C Mater Biol Appl. 2019. PMID: 31500051
-
Polycaprolactone- and polycaprolactone/ceramic-based 3D-bioplotted porous scaffolds for bone regeneration: A comparative study.Mater Sci Eng C Mater Biol Appl. 2017 Oct 1;79:326-335. doi: 10.1016/j.msec.2017.05.003. Epub 2017 May 4. Mater Sci Eng C Mater Biol Appl. 2017. PMID: 28629025
-
Fabrication of Mechanically Reinforced Gelatin/Hydroxyapatite Bio-Composite Scaffolds by Core/Shell Nozzle Printing for Bone Tissue Engineering.Int J Mol Sci. 2020 May 11;21(9):3401. doi: 10.3390/ijms21093401. Int J Mol Sci. 2020. PMID: 32403422 Free PMC article.
-
Fabrication techniques involved in developing the composite scaffolds PCL/HA nanoparticles for bone tissue engineering applications.J Mater Sci Mater Med. 2021 Aug 11;32(8):93. doi: 10.1007/s10856-021-06564-0. J Mater Sci Mater Med. 2021. PMID: 34379204 Free PMC article. Review.
Cited by
-
Silver Nanoparticles in 3D Printing: A New Frontier in Wound Healing.ACS Omega. 2024 Sep 16;9(40):41107-41129. doi: 10.1021/acsomega.4c04961. eCollection 2024 Oct 8. ACS Omega. 2024. PMID: 39398164 Free PMC article. Review.
-
New Generation of Osteoinductive and Antimicrobial Polycaprolactone-Based Scaffolds in Bone Tissue Engineering: A Review.Polymers (Basel). 2024 Jun 12;16(12):1668. doi: 10.3390/polym16121668. Polymers (Basel). 2024. PMID: 38932017 Free PMC article. Review.
-
Towards Polycaprolactone-Based Scaffolds for Alveolar Bone Tissue Engineering: A Biomimetic Approach in a 3D Printing Technique.Int J Mol Sci. 2023 Nov 10;24(22):16180. doi: 10.3390/ijms242216180. Int J Mol Sci. 2023. PMID: 38003368 Free PMC article. Review.
-
Trends in bioactivity: inducing and detecting mineralization of regenerative polymeric scaffolds.J Mater Chem B. 2024 Mar 13;12(11):2720-2736. doi: 10.1039/d3tb02674d. J Mater Chem B. 2024. PMID: 38410921 Free PMC article. Review.
-
Additive manufacturing of poly (lactic acid)/hydroxyapatite/carbon nanotubes biocomposites for fibroblast cell proliferation.Sci Rep. 2023 Nov 21;13(1):20387. doi: 10.1038/s41598-023-47413-0. Sci Rep. 2023. PMID: 37990057 Free PMC article.
References
-
- Rodriguez G, et al. Influence of hydroxyapatite on extruded 3D scaffolds. Procedia Eng. 2013;59:263–9. doi: 10.1016/j.proeng.2013.05.120. - DOI
-
- Eshraghi S, Das S. Mechanical and microstructural properties of polycaprolactone scaffolds with one-dimensional, two-dimensional, and three-dimensional orthogonally oriented porous architectures produced by selective laser sintering. Acta biomaterialia. 2010;6:2467–76. doi: 10.1016/j.actbio.2010.02.002. - DOI - PMC - PubMed
MeSH terms
Substances
LinkOut - more resources
Full Text Sources