3D printed devices and infrastructure for liquid sample delivery at the European XFEL
- PMID: 35254295
- PMCID: PMC8900844
- DOI: 10.1107/S1600577521013370
3D printed devices and infrastructure for liquid sample delivery at the European XFEL
Abstract
The Sample Environment and Characterization (SEC) group of the European X-ray Free-Electron Laser (EuXFEL) develops sample delivery systems for the various scientific instruments, including systems for the injection of liquid samples that enable serial femtosecond X-ray crystallography (SFX) and single-particle imaging (SPI) experiments, among others. For rapid prototyping of various device types and materials, sub-micrometre precision 3D printers are used to address the specific experimental conditions of SFX and SPI by providing a large number of devices with reliable performance. This work presents the current pool of 3D printed liquid sample delivery devices, based on the two-photon polymerization (2PP) technique. These devices encompass gas dynamic virtual nozzles (GDVNs), mixing-GDVNs, high-viscosity extruders (HVEs) and electrospray conical capillary tips (CCTs) with highly reproducible geometric features that are suitable for time-resolved SFX and SPI experiments at XFEL facilities. Liquid sample injection setups and infrastructure on the Single Particles, Clusters, and Biomolecules and Serial Femtosecond Crystallography (SPB/SFX) instrument are described, this being the instrument which is designated for biological structure determination at the EuXFEL.
Keywords: FEL physics; X-ray scattering; aerosols; crystallography; high-viscosity extrusion; instrumentation; liquid jets; microfluidics; rapid mixing; sample delivery; single-particle imaging.
open access.
Figures
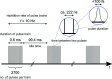
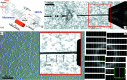
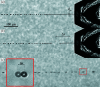
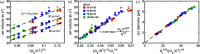
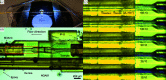
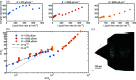
Similar articles
-
Microfluidic polyimide gas dynamic virtual nozzles for serial crystallography.Rev Sci Instrum. 2020 Aug 1;91(8):085108. doi: 10.1063/5.0012806. Rev Sci Instrum. 2020. PMID: 32872940
-
A versatile liquid-jet setup for the European XFEL.J Synchrotron Radiat. 2019 Mar 1;26(Pt 2):339-345. doi: 10.1107/S1600577519000894. Epub 2019 Feb 22. J Synchrotron Radiat. 2019. PMID: 30855241 Free PMC article.
-
Pump-probe capabilities at the SPB/SFX instrument of the European XFEL.J Synchrotron Radiat. 2022 Sep 1;29(Pt 5):1273-1283. doi: 10.1107/S1600577522006701. Epub 2022 Jul 21. J Synchrotron Radiat. 2022. PMID: 36073887 Free PMC article.
-
Sample delivery for serial crystallography at free-electron lasers and synchrotrons.Acta Crystallogr D Struct Biol. 2019 Feb 1;75(Pt 2):178-191. doi: 10.1107/S205979831801567X. Epub 2019 Jan 9. Acta Crystallogr D Struct Biol. 2019. PMID: 30821706 Free PMC article. Review.
-
Dynamic Structural Biology Experiments at XFEL or Synchrotron Sources.Methods Mol Biol. 2021;2305:203-228. doi: 10.1007/978-1-0716-1406-8_11. Methods Mol Biol. 2021. PMID: 33950392 Review.
Cited by
-
A sub-100 nm thickness flat jet for extreme ultraviolet to soft X-ray absorption spectroscopy.J Synchrotron Radiat. 2024 May 1;31(Pt 3):605-612. doi: 10.1107/S1600577524001875. Epub 2024 Apr 9. J Synchrotron Radiat. 2024. PMID: 38592969 Free PMC article.
-
3D-printed sheet jet for stable megahertz liquid sample delivery at X-ray free-electron lasers.IUCrJ. 2023 Nov 1;10(Pt 6):662-670. doi: 10.1107/S2052252523007972. IUCrJ. 2023. PMID: 37721770 Free PMC article.
-
A multi-million image Serial Femtosecond Crystallography dataset collected at the European XFEL.Sci Data. 2022 Apr 12;9(1):161. doi: 10.1038/s41597-022-01266-w. Sci Data. 2022. PMID: 35414146 Free PMC article.
-
Thinner than a knife's edge: 3D-printed liquid sheet jet technology for solution phase XFEL experiments.IUCrJ. 2023 Nov 1;10(Pt 6):638-641. doi: 10.1107/S2052252523009429. IUCrJ. 2023. PMID: 37910141 Free PMC article.
-
A snapshot love story: what serial crystallography has done and will do for us.Acta Crystallogr D Struct Biol. 2024 Aug 1;80(Pt 8):563-579. doi: 10.1107/S2059798324005588. Epub 2024 Jul 10. Acta Crystallogr D Struct Biol. 2024. PMID: 38984902 Free PMC article. Review.
References
-
- Allahgholi, A., Becker, J., Delfs, A., Dinapoli, R., Goettlicher, P., Greiffenberg, D., Henrich, B., Hirsemann, H., Kuhn, M., Klanner, R., Klyuev, A., Krueger, H., Lange, S., Laurus, T., Marras, A., Mezza, D., Mozzanica, A., Niemann, M., Poehlsen, J., Schwandt, J., Sheviakov, I., Shi, X., Smoljanin, S., Steffen, L., Sztuk-Dambietz, J., Trunk, U., Xia, Q., Zeribi, M., Zhang, J., Zimmer, M., Schmitt, B. & Graafsma, H. (2019). J. Synchrotron Rad. 26, 74–82. - PMC - PubMed
-
- Bean, R. J., Aquila, A., Samoylova, L. & Mancuso, A. P. (2016). J. Opt. 18, 074011.
-
- Bernardeschi, I., Ilyas, M. & Beccai, L. (2021). Adv. Intell. Syst. 3, 2100051.
-
- Beyerlein, K. R., Adriano, L., Heymann, M., Kirian, R., Knoška, J., Wilde, F., Chapman, H. N. & Bajt, S. (2015). Rev. Sci. Instrum. 86, 125104. - PubMed
-
- Beyerlein, K. R., Dierksmeyer, D., Mariani, V., Kuhn, M., Sarrou, I., Ottaviano, A., Awel, S., Knoska, J., Fuglerud, S., Jönsson, O., Stern, S., Wiedorn, M. O., Yefanov, O., Adriano, L., Bean, R., Burkhardt, A., Fischer, P., Heymann, M., Horke, D. A., Jungnickel, K. E. J., Kovaleva, E., Lorbeer, O., Metz, M., Meyer, J., Morgan, A., Pande, K., Panneerselvam, S., Seuring, C., Tolstikova, A., Lieske, J., Aplin, S., Roessle, M., White, T. A., Chapman, H. N., Meents, A. & Oberthuer, D. (2017). IUCrJ, 4, 769–777. - PMC - PubMed
MeSH terms
Grants and funding
LinkOut - more resources
Full Text Sources